AZoLifeSciences talks to Dr. Gerald Meehl about how recent climate models have shown increased sensitivity to CO2, how cloud processes could be affecting these models, and what this means for climate warming in the future.
What instigated this research?
Some of the most recent climate models were showing higher than expected values of something called “equilibrium climate sensitivity” (ECS) which is a hypothetical value of how much the earth would eventually warm after atmospheric carbon dioxide was doubled. But the overall range of ECS has increased, both higher at the high end and lower at the low end, compared to previous generations of models.
We wanted to see if we could identify the factors that were producing this increased range of responses.
How do climate models influence our knowledge about climate change and why are they so important?
Climate models are the only tools we have to estimate how the climate will change in the future. They encapsulate the state-of-the-science and represent the current level of human understanding of climate variability and climate change.
Therefore, the more credible the model, measured by the fidelity of how well the model can simulate the climate we have already observed, the more credible its estimates of future changes will be.
What do recent climate models suggest about the future?
Climate models are typically run with different possible scenarios of future emissions of greenhouse gases. Some scenarios are optimistic and presume human societies will be able to reduce emissions, while other scenarios are less optimistic.
The range of these possible future responses, dependent on when and how much we can reduce greenhouse gas emissions, indicate the levels of future warming we may experience, with associated impacts on societies, infrastructure, and ecosystems.
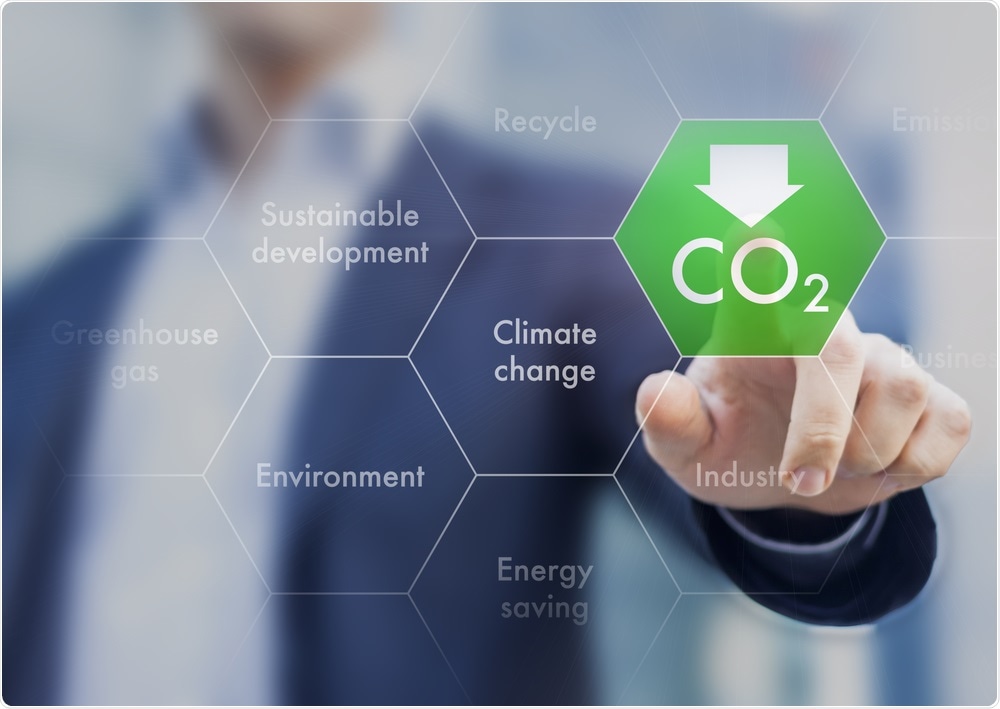
Image Credit: NicoElNino/Shutterstock.com
How have these recent models shown higher sensitivity to CO2 and subsequently increased warming?
We showed that recent models with higher values of ECS have larger contributions from cloud feedbacks, such that changes in clouds produce higher levels of warming in those models.
However, some models with ECS on the low end also have contributions from cloud feedbacks.
This highlights the important role of cloud feedbacks in the climate model responses to increasing greenhouse gases.
How could updates to modern climate models be causing or exposing errors?
The cloud formulations in the current climate models are more complex and therefore more realistic. This has improved the simulation of clouds in the models. However, several interacting cloud processes are now included in the models that contribute to increasing the uncertainty in some of the feedbacks.
For example, many models now include interactions between visible air pollution (atmospheric aerosols) and clouds. The more polluted the air, the more aerosol particles are available for cloud condensation nuclei. This results in more but smaller cloud droplets, and this produces brighter clouds that reflect more sunlight and cause a cooling effect.
However, some aerosols absorb sunlight, warm the air, and thus contribute to evaporating some cloud droplets.
This results in fewer clouds and allows more sunlight to reach the ground which is a warming effect. The size and nature of these different feedbacks, interacting with other feedbacks in the system, contribute to a larger range of ECS.
What is the relationship between clouds and climate warming in the real world?
This is one of the most challenging questions in climate science because, averaged over the whole globe for all kinds of cloud types at different altitudes and locations, if the net effect of clouds is to warm the system, then cloud feedbacks could contribute to enhanced warming.
Conversely, if the net effect of all those clouds is to cool the system, then they would act to reduce warming. Understanding how clouds function in the system requires enhanced observations from satellites and the surface.
It is only with better observations that we can improve our understanding, and that improved understanding is then reflected in improved climate models that produce more credible projections of the future climate.
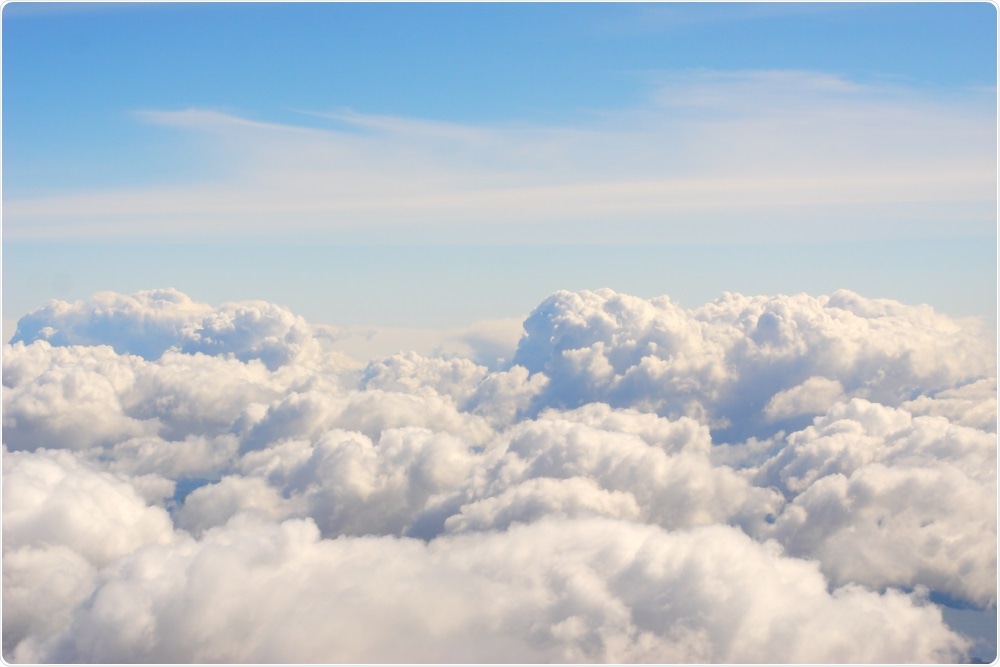
Image Credit: Genova/Shutterstock.com
How did you go about analyzing the models to identify this possible reason?
Models are compared to all available observations to see how well they are able to simulate those observations. If there are differences between the model and observations, then the model formulations must be improved, though, as noted above, it is difficult to model what we do not understand.
In the end, the limitations come down to how well we understand how clouds behave in the real system.
There are different methods by which researchers evaluate climate model sensitivity.
What are the ECS and TCR metrics and how do they compare?
ECS is the hypothetical warming at equilibrium (a state that is never achieved in the climate system) for a doubling of atmospheric carbon dioxide. This is a historical measure of climate system response that arose from the earliest studies of the energy balance of the system. This metric is typically computed for each new model version to compare to previous generations of models.
However, ECS can never be observed, even though it can be calculated in a numerical model. The TCR is more relevant for multi-decadal timescales since it is computed in an experiment where CO2 is increased 1% per year compounded, and when CO2 reaches twice its present-day value (around year 70 in such an idealized experiment), the global surface temperature increase is calculated.
The range and average values of TCR have remained more constant over generations of climate models, including the present CMIP6 models.
This indicates that the long-term feedbacks that contribute to the eventual values of ECS do not all kick in during the first 70 years of an experiment where TCR is calculated. Many scientists argue that TCR is the more relevant metric to compare model responses to increasing CO2 on timescales of less than a century.
Considering your findings and how you think these models are being affected, what do you think is the likely trajectory for climate warming over the next few decades?
There is quite good agreement among the models regarding warming over the next few decades for several reasons.
First, the response to the different possible future emission scenarios does not diverge until about mid-century.
Second, the feedbacks that contribute to the smaller range and more stable response of TCR over time that work on multi-decadal timescales indicate that there should be better model agreement over the next few decades. Going to the end of the century and beyond, uncertainty grows from the different scenario outcomes and the longer-term feedbacks that produce a larger range of ECS compared to TCR.
What are the next steps for your research?
Cloud feedbacks in general, and cloud-aerosol interactions in particular, are high priorities for the climate science community.
Where can readers find more information?
- Meehl, G.A., C.A. Senior, V. Eyring, G. Flato, J.-F. Lamarque, R.J. Stouffer, K.E. Taylor, and M. Schlund, 2020: Context for interpreting equilibrium climate sensitivity and transient climate response from the CMIP6 Earth system models, Science Advances, doi:10.1126/sciadv.aba1981.
About Dr. Gerald. A. Meehl
Gerald A. Meehl is a Senior Scientist at the National Center for Atmospheric Research (NCAR) and heads the Climate Change Research Section. He is also Chief Scientist of the Cooperative Agreement to Analyze variability, change, and predictability in the earth System (CATALYST), a project that involves NCAR and the U.S. Department of Energy (DOE).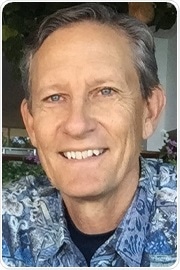
His research interests include studying the interactions between El Niño/Southern Oscillation (ENSO) and the monsoons of Asia; identifying possible effects on global climate of changing anthropogenic forcings, such as carbon dioxide, as well as natural forcings, such as solar variability; quantifying possible future changes of weather and climate extremes in a warmer climate; and understanding the interplay between internally generated climate variability and the response to external forcings, particularly in the context of understanding and predicting decadal climate variability.
He was a member of the Intergovernmental Panel on Climate Change (IPCC) science team that was awarded the Nobel Peace Prize in 2007. As part of that effort, he was involved with the first five IPCC climate change assessment reports, serving as contributing author (1990), lead author (1995), coordinating lead author (2001, 2007), and lead author on the near-term climate change chapter for the IPCC AR5 that was completed in 2013.
He received his Ph.D. in climate dynamics from the University of Colorado and was a recipient of the Jule G. Charney Award of the American Meteorological Society in 2009. Dr. Meehl is an Associate Editor for the Journal of Climate, a Fellow of both the American Meteorological Society and the American Geophysical Union, and a Visiting Senior Fellow at the University of Hawaii Joint Institute for Marine and Atmospheric Research.
He serves as co-chair of the Community Earth System Model Climate Variability and Change Working Group, and is a member of the DOE Biological and Environmental Research Advisory Committee (BERAC).
Additionally, he has been a member and chair of the National Academy of Sciences Climate Research Committee (CRC), co-chair of the National Academy of Sciences Board on Atmospheric Sciences and Climate (BASC), member and co-chair of the World Climate Research Programme (WCRP) Working Group on Coupled Models (WGCM, the group that coordinates the international global climate model experiments addressing anthropogenic climate change through the Coupled Model Intercomparison Project, CMIP), and is currently co-chair of the WCRP Model Advisory Council (WMAC).
He also is a member of the WGCM CMIP Panel that is tasked with coordinating the current CMIP6 international model intercomparison activity.