Vibrational spectroscopy comprises a range of techniques that detects molecular vibrations in samples. Generally, these techniques include Raman and infrared spectroscopy, which are complementary techniques used to elucidate the molecular structure and understand intra/intermolecular local environments.
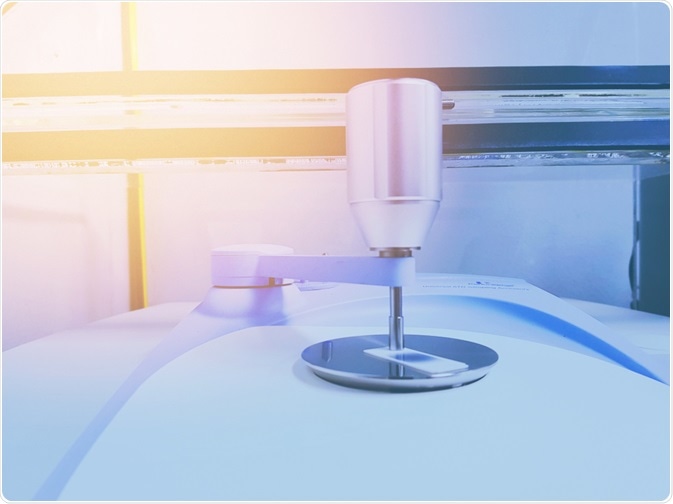
Image Credit: Ken stocker/Shutterstock.com
This is because molecular vibrations are extremely sensitive to environmental conditions, temperature, and various molecular interactions. The demand for fast non-invasive analysis of different physicochemical properties of molecules with accurate quantitative and qualitative analysis allows for a wide range of applications using vibrational spectroscopic techniques.
This has led to the use of Raman and infrared spectroscopy as preferred techniques over more time-consuming analytical techniques such as chromatography (gas chromatography (GC), high-performance liquid chromatography (HPLC)), mass spectrometry and nuclear magnetic resonance (NMR).
What is Vibrational Spectroscopy?
Changes in the energy of atoms and molecules as they transition between distinct energy levels cause them to emit or absorb electromagnetic radiation. Different energy transitions result in different frequencies of electromagnetic radiation.
The basic principle of spectroscopy is to irradiate molecules in a sample with a select portion of the electromagnetic spectrum to observe the interaction of radiation with the sample. Every molecule has a distinctive pattern of absorption and emission of radiation.
A molecular vibration occurs when atoms in a molecule are in periodic motion relative to the constant transitional and rotational motion of the entire molecule. Thus, the vibrational frequency is the frequency of the periodic motion.
For a molecule with N atoms, there are 3N – 6 vibrational modes for non-linear molecules and 3N – 5 for linear molecules. Hence, when the number of atoms increases so does the number of corresponding vibrational modes.
Vibrational motions can be either stretching or bending vibrational modes. A stretching vibration involves a continuous change in the interatomic distance along the axis of the bond between two atoms. A bending vibration (or deformation mode) is when there is a change in the angle between two bonds.
Infrared and Raman spectroscopy are techniques in vibrational spectroscopy that exploit these molecular motions.
Infrared Spectroscopy
Infrared spectroscopy is an absorption technique that uses the infrared portion of the electromagnetic spectrum, which includes near-IR (NIR), mid-IR (MIR), and far IR (also known as terahertz). The near-IR region is approximately 14000 – 4000 cm−1 and can excite overtone or combination modes of molecular vibrations.
The mid-infrared region is approximately 4000 – 200 cm−1 and is generally used to study the fundamental vibrations (fingerprint region). The far-infrared or terahertz region is approximately 200 – 10 cm−1 and may be used for rotational spectroscopy and low-frequency vibrations. Infrared absorption occurs when a vibrational mode of a molecule has a net change in dipole moment where radiation of the same frequency interacts with it and causes it to be promoted to an excited vibrational state.
Different types of infrared spectroscopy have been used for various applications in life science, bioscience, biomedical diagnostics, and environmental science. Commonly, Fourier Transform algorithms are used in conjunction with infrared to produce FTIR instrumentation.
Traditionally, dispersive IR spectrometers could only access small portions of the spectral region at any given time, which resulted in the slow acquisition of data. However, modern FTIR spectrometers have the advantage of the detector simultaneously accessing all frequencies generated by the radiation source. Thus, giving them much faster acquisition times.
FTIR spectroscopic techniques have a broad range of applications especially in biomedical diagnostics of cancer, disease, and infections. Some applications use FTIR with synchrotron radiation, focal plane array detectors (FPA) and attenuated total reflectance (ATR) to detect malarial infections.
For example, these techniques were used to accurately detect metabolized hemoglobin in the digestive vacuole of the malarial parasite (hemozoin) as well as accurately diagnosing the different lifecycle stages of the malarial parasite based on a change in fatty acid composition and presence of hemozoin within a red blood cell.
Other applications have exploited the use of diffuse reflectance FTIR (DRIFT) in environmental science to detect soil properties and various contaminants in the soil for commercial applications. Other applications using NIR have been employed to detect various sugars and acidity in apples.
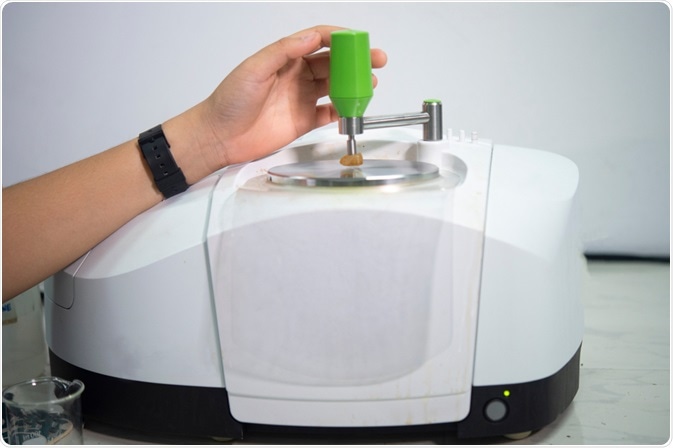
Image Credit: Rabbitmindphoto/Shutterstock.com
Raman Spectroscopy
In contrast, Raman spectroscopy is a scattering technique and is complementary to infrared spectroscopy. It uses the effect of inelastic scattering of monochromatic light to produce the Raman effect. The intensity of Raman scattering is inversely proportional to the wavelength of light.
Hence, a shorter wavelength will produce a larger Raman effect than longer wavelengths. There are different types of scattering mechanisms. One of which is resonance Raman scattering, which involves the enhancement of Raman intensities by exciting molecules at resonance or pre-resonance with wavelengths that undergo electronic transitions.
By tuning into different electronic absorption bands, vibrational modes can be selectively enhanced. One such application that exploited this mechanism was to investigate structural changes in haem proteins. An advantage of Raman over infrared is that water does not have an interference in the spectrum like infrared.
Thus, Raman spectroscopy has wide applications in biological sciences and biomedical diagnostics for studying biological samples as well as various infections.
Another technique used in vibrational spectroscopy is surface-enhanced Raman scattering (SERS), which is a surface enhancement technique that increases the Raman signal on metal surfaces by a surface plasmon with metallic thin-film structures.
In contrast, a less sensitive technique is spatially offset Raman spectroscopy (SORS). However, it has the added advantage of detecting drugs without opening packaging and to non-invasively analyze biological tissue.
Moreover, another vibrational spectroscopy technique is Raman micro-spectroscopy that uses microscopes to analyze and detect various molecules in biological samples such as subcellular structures in cells.
Advancements in complementary vibrational spectroscopy (CVS) combines IR and Raman spectroscopy as a dual-modal approach for simultaneously obtaining IR and Raman spectra. This approach gives the potential for studying dynamic change in complex molecular structures at a given measurement position in the sample.
This has a variety of applications for determining molecular symmetry or for two-dimensional correlation spectroscopy. Thus, advances in chemometrics and multivariate statistics combined with advanced techniques of infrared and Raman spectroscopy give broad applications for vibrational spectroscopy.
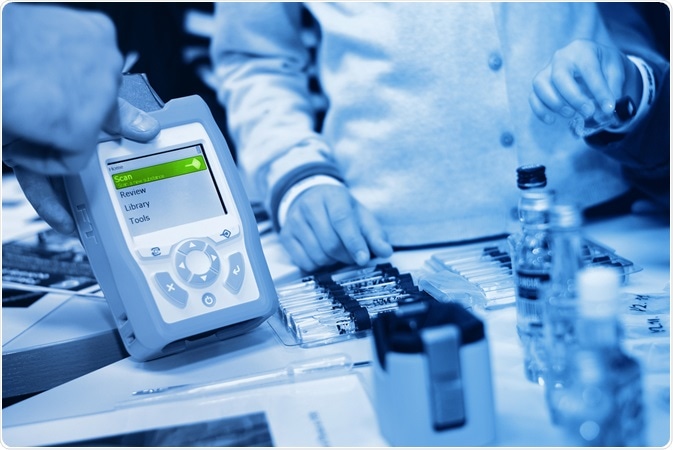
Image Credit: Forance/Shutterstock.com
References
- Pezzei, C.K.; Watschinger, M.; Huck-Pezzei, V.A.; Lau, C.; Zuo, Z.; Leung, P.C.; Huck, C.W. “Infrared spectroscopic techniques for the non-invasive and rapid quality control of Chinese traditional medicine”. Si-Wu-Tang. Spectrosc. Eur. 2016, 28, 16–21.
- Siesler, H.W.; Ozaki, Y.; Kawata, S.; Heise, H.M.; (Eds.) Near-Infrared Spectroscopy: Principles, Instruments, Applications; Wiley: Weinheim, Germany, 2008.
- Sasic, S.; Ozaki, Y. Raman, Infrared, and Near-Infrared Chemical Imaging; Wiley: Hoboken, NJ, USA, 2010.
- Zeitler, J. Axel; Taday, Philip F.; Newnham, David A.; Pepper, Michael; Gordon, Keith C.; Rades, Thomas (2007). "Terahertz pulsed spectroscopy and imaging in the pharmaceutical setting - a review". Journal of Pharmacy and Pharmacology. 59 (2): 209–223.
- Atkins PW, de Paula J (2009). Elements of physical chemistry (5th ed.). Oxford: Oxford U.P. p. 459.
- Webster, G. T., de Villiers, K., Egan, T. J., Deed, S., Tilley, L., Tobin, M., Bambery, K., McNaughton, D., Wood, B. R. J. Anal. Chem. 2009, 81, 2516-2524.
- Webster, G. T, Soriano-Disla, J. M, Kirk, J, Janik, L. J, Forrester, S. T, McLaughlin, M. J, Stewart, R. J, Talanta. 2017, 160, 410–416.
- Eisenstecken, D.; Panarese, A.; Robatscher, P.; Huck, C.; Zanella, A.; Oberhuber, M. A Near-Infrared Spectroscopy (NIRS) and Chemometric Approach to Improve Apple Fruit Quality Management: A Case Study on the Cultivars “Cripps Pink” and “Braeburn”. Molecules 2015, 20, 13603 – 13619.
- Webster, G. T., McNaughton, D., Wood, B. R. J. Phys. Chem. B. 2009, 113, 6910-6916.
- Wood, B. R., Hermelink, A., Lasch, P., Bambery. K. R., Webster, G. T., Asghari Khiavi, M., Cooke, B. M., Deed, S., Naumann, D., McNaughton, D. Analyst. 2009, 134, 1119-1125.
- Webster, G. T, Tilley, L, Deed, S, McNaughton, D, Wood, B. R, FEBS Lett. 2008, 582, 1087-1092.
- Skoog, D. A.; Holler, E. J.; Nieman, T. A. Principles of Instrumental Analysis 5th Edition; Saunders College Publishing: USA, 1992.
- Spiro, T. G.; Strekas, T. J. Am. Chem. Soc. 1974, 96, 338 – 345.
- Spiro, T. G.; Strekas, T. Proc. Nat. Acad. Sci. USA. 1972, 69, 2622 – 2626.
- Butler, Holly J.; Ashton, Lorna; Bird, Benjamin; Cinque, Gianfelice; Curtis, Kelly; Dorney, Jennifer; Esmonde-White, Karen; Fullwood, Nigel J.; Gardner, Benjamin; Martin-Hirsch, Pierre L.; Walsh, Michael J.; McAinsh, Martin R.; Stone, Nicholas; Martin, Francis L. (2016). "Using Raman spectroscopy to characterize biological materials". Nature Protocols. 11 (4): 664–687.
- Taylor, P.D.; Vinn, O.; Kudryavtsev, A.; Schopf, J.W. (2010). "Raman spectroscopic study of the mineral composition of cirratulid tubes (Annelida, Polychaeta)". Journal of Structural Biology. 171 (3): 402–405.
- Blackie, Evan J.; Le Ru, Eric C.; Etchegoin, Pablo G. (2009). "Single-Molecule Surface-Enhanced Raman Spectroscopy of Nonresonant Molecules". J. Am. Chem. Soc. 131 (40): 14466–14472.
- Müller, N., Brückner, L. & Motzkus, M. Coherent Raman and mid-IR microscopy using shaped pulses in a single-beam setup. APL Photonics 3, 092406 (2018).
- Ziffer, H. & Levin, I. W. Determining centrosymmetric dimers by infrared and Raman spectroscopy. J. Org. Chem. 34, 4056–4060 (1969).
- Barón, M., Bain, A. D. & Conde, R. S. A dynamic look on molecular symmetry. Can. J. Chem. 95, 736–743 (2017).
- Park, Y., Noda, I. & Jung, Y. M. Two-dimensional correlation spectroscopy in polymer study. Front. Chem. 3, 14 (2015).
Further Reading