There is a growing need to develop highly sensitive and accurate instruments capable of detecting and analyzing trace explosives due to the steady increase in terrorist attacks worldwide.
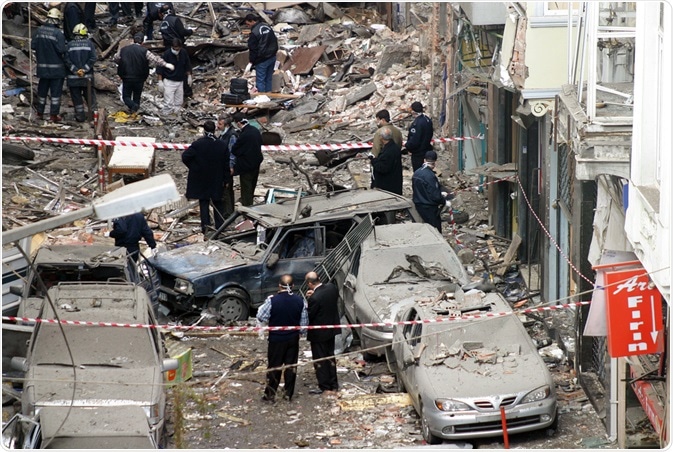
Image Credit: Prometheus72/Shutterstock.com
Recent advances in mass spectrometry, which has already been applied to several forensic cases, have supported its usefulness as a security tool for the analysis of explosives.
Mass spectrometry in forensics
The basic principle of any mass spectrometry (MS) analysis is to identify the time and space of the path of a charged particle, which is dependent upon the particle’s mass-to-charge ratio (m/z) as it passes through an electromagnetic field in a vacuum.
Several different types of MS have proven to be vital tools for several decades within the field of forensic science. Some of the earliest applications of MS in forensics occurred during the 1950s and 1960s when this analytical tool was used to characterize the elemental compositions of natural products and drugs.
Further utilization of this technique took place during the 1970s, during which MS, particularly when coupled with gas chromatography (GC-MS), allowed for forensic scientists to confirm the presence of drugs of abuse as well as assist in forensic toxicology studies.
Ambient MS
Despite its usefulness for the identification of chemical compounds present within a mixture, MS is associated with a significant limitation for researchers and their samples.
To this end, MS users are required to insert their sample of interest, which must be in its ionic form, into the vacuum environment of the spectrometer for mass analysis to occur.
Electrospray ionization (ESI), which involves the nebulization of a chemical mixture to create a fine spray of droplets, has improved the ability of researchers to transform their chemical solutions into molecular ions that can be suitable for the analyzer.
Aside from incorporating ESI to allow chemical mixtures to be suitable for vacuum conditions, ions can also be generated in air. Ambient MS experiments, in which chemical species are ionized and sampled under ambient conditions, have been shown to allow a fast analysis of untreated surfaces in their native environment without compromising its chemical specificity and detection limits.
Some of the most recent applications of ambient MS in forensics include the analysis of drug metabolites in urine, lipids within intact tissues, active ingredients in pharmaceutical tablets and the detection of trace explosives on luggage.
Limitations of traditional trace explosive detection methods
Traditionally, national security officials, of which include the national defense, customs, and border control, as well as those managing transportation security, have utilized ion mobility spectrometry (IMS) and colorimetric techniques to rapidly characterize trace explosives.
Despite the portability of these devices, they have often been associated with poor specificity, thereby limiting their usefulness during emergencies.
Additional challenges that are associated with the detection of trace explosives are the fact that these compounds may be present in a wide range of chemical forms like particulate material, residue, liquid, emulsion, vapor or aerosol species. Therefore, it is crucial that the analytical technique that is used to identify trace explosives is not only highly sensitive and specific but is also flexible in its capability to analyze various sample mediums.
Detecting explosives by ambient MS
As compared to IMS and colorimetric techniques, MS techniques, particularly GC-MS, liquid chromatography MS (LC-MS) and tandem MS have demonstrated their superior sensitivity and specificity capabilities for a wide range of sample types.
Since GC-MS, LC-MS, and tandem MS are associated with certain limitations in terms of extensive sample preparation requirements and longer run times, ambient MS has emerged as an ideal tool for the analysis of trace explosives.
With little to no sample preparation required and its rapid analytical capabilities, ambient MS has remained as an integral aspect of explosive detection for over 10 years.
In addition to its inherent advantages, such as its ability to directly couple to various miniature and field-portable MS devices, ambient MS has demonstrated its usefulness to provide highly sensitive and specific data on-site during the detection of trace explosives.
Current applications
Organic nitrate-based explosives, of which include nitroaromatic, nitramine and nitrate ester explosives, are the most widely studied in general explosive detection studies, as well as those involving ambient MS.
The most notable ambient MS platform includes desorption electrospray ionization (DESI), which has successfully been used to detect explosives present on paper, plastic, stainless steel, glass, nitrile gloves, skin and a wide range of fabric surfaces.
By utilizing this technology, security officials can directly analyze materials, particularly fabrics, thereby eliminating the need to swab or dust these materials. This ultimately eliminates any potential degradation of the target analytes that would otherwise occur during swab transfers, or as a result of the longer wait times between sample collection and its analysis.
Aside from criminal investigations, DESI coupled ambient MS also has the potential to assist in quality control and testing of personal protective equipment (PPE) to quickly and thoroughly assess the safety of workers' emergencies, such as chemical spills.
References
- Jackson, G.P. and M.A. Barkett, A History of the Forensic Applications of Mass Spectrometry. The Encylopedia of Mass Spectrometry Volume 9: Historical Perspectives, Part A: The Development of Mass Spectrometry. Vol. 9. 2016: Elsevier.
- Cooks, R.G., et al., Science, 2006. 311: p. 1566 - 1570.
- Venter, A., M. Nefliu, and R.G. Cooks, TrAC, Trends Anal. Chem., 2008. 27: p. 284 - 290.
- Forbes, T.P. and E. Sisco, Recent advances in ambient mass spectrometry of trace explosives. Analyst, 2018. 9(143): p. 1948-1969.
- H, L. and K.S. Suslick, J. Am. Chem. Soc., 2010. 132: p. 15519 - 15521.
- Peng, Y., et al., Chem. Commun., 2011. 47: p. 4505 - 4507.
- Peters, K.L., et al., Anal. Methods, 2015. 7: p. 63 - 70.
- Bottegal, M., et al., Rapid Commun. Mass Spectrom, 2010. 24: p. 1377-1386.
- Halasz, A., et al., J. Chromatogr, A, 2002. 963: p. 411 - 418.
- McEwen, C.N., R.G. McKay, and B.S. Larsen, Anal. Chem., 2005. 77: p. 7826-7831.
- Perret, D., et al., Chromatographia, 2008. 68: p. 517-524.
- Justes, D.R., et al., Chem. Commun., 2007: p. 2142 - 2144.
- Talaty, N., Z. Takats, and R.G. Cooks, Analyst, 2005. 130: p. 1624 - 1633.
Further Reading