Bioinformatics uses sensors and computers to capture, track, analyze, and interpret biological data, with extensive applications in any field with large data sets, such as DNA sequencing or proteomics. Wearable sensors are set to provide a huge quantity of data relating to health and physical condition, from heart rate to blood glucose levels, providing the opportunity to monitor and predict physical function and disease occurrence.
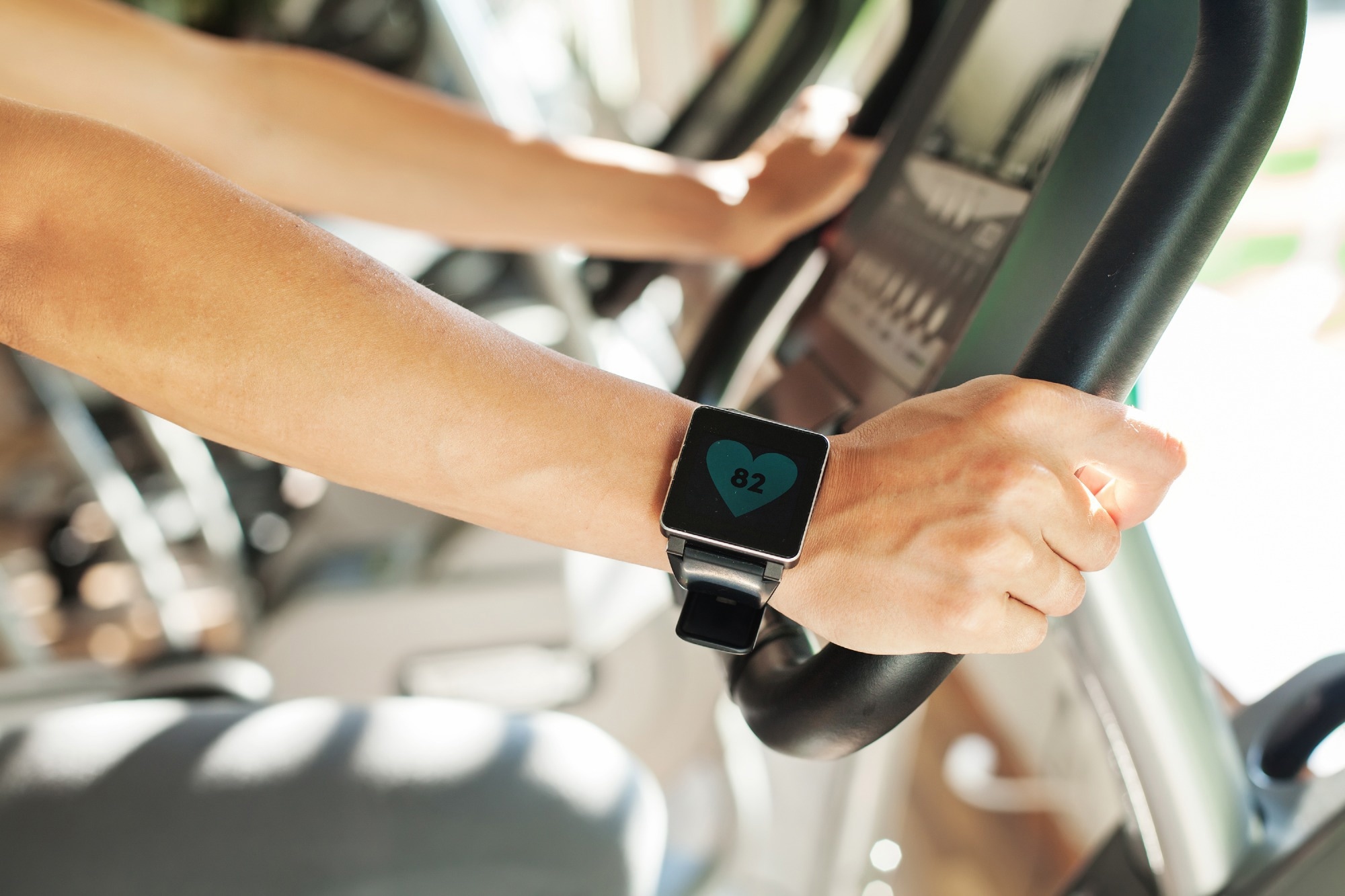
Image Credit: Mila Supinskaya Glashchenko/Shutterstock.com
Therefore, bioinformatics application using wearable sensors depends on innovation in numerous fields, from the hardware that interfaces with the human wearer to the software that interprets and outputs analysis. This article will briefly discuss the current progress and future prospects of wearable technology and the importance of bioinformatics in using this vast quantity of data.
What are the medical applications of wearable sensors?
In 1903 the world's first electrocardiograph was invented by William Einthoven to measure the electrical activity of the heart, and since this time, great strides have been made in electrical measurement. A biosensor consists of three components: the recognition element, which detects electrical or chemical signals; the transducer, which converts this into an analog electrical signal; and an acquisition system, which digitizes and records the data.
One of the most explored applications of biosensors is in glucose monitoring for those with diabetes, and in this case, the detector typically operates by measuring the quantity of molecular oxygen, hydrogen peroxide, or other chemicals associated with glucose metabolism. Continuous subdermal glucose monitoring has been achieved using small needle-like sensors based on changing potential across electrodes in the presence of glucose, though less invasive continuous monitoring has been more difficult to achieve.
For example, the GlucoWatch operated by reverse iontophoresis of interstitial fluid to perform transdermal glucose sensing but was withdrawn from the market in 2008 owing to skin irritation and problems with accuracy. Modern devices generally incorporate wireless transmission technology to allow regular data packets to be transmitted to the user or physicians and to provide real-time alerts if blood sugar levels spike or lower dangerously.
Simple applications such as this are the immediate concern of wearable medical sensors, with bioinformatics utilized to gather data relating to wide sections of the population and subsequently refine the function and operation of such devices in successive generations. Wearable or implanted biosensors are capable of collecting data relating to a huge range of parameters that must be sorted and concurrently interpreted in real-time for the greatest value, and initially identifying how these parameters actually relate to human health is perfectly within the realm of application for machine learning algorithms, as have been utilized extensively in the field of genomics.
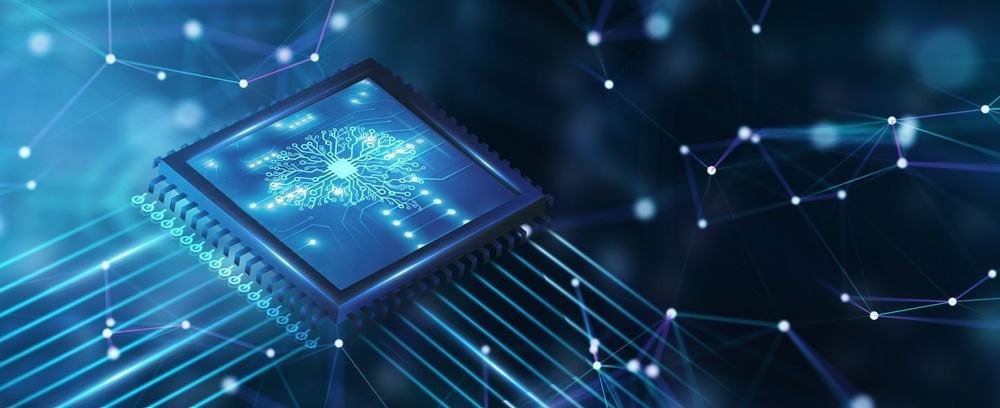
Image Credit: Den Rise/Shutterstock.com
Why is machine learning required to interpret data from wearable sensors?
Bioinformatics is important in developing complex prosthetics, such as retinal prostheses, for treating degenerative retinal diseases. Mathieson et al. (2012) developed a retinal prosthesis to replace the eye's non-functioning "image capture" region and interact with the still functioning image processing neurons of the inner eye.
The device operates using an array of photodiodes that provide electrical stimulation to neurons when struck with light, and the group demonstrated neuronal activity in enucleated rat eyes with a healthy or degenerated retina that suggests a further refinement of the prosthesis could allow practical use in humans in the future. The potential of such technology relies on bioinformatics to collect, sort, refine, and interpret results, often utilizing machine learning, data mining techniques, and artificial intelligence to automate these processes and spot trends outside the scope of a human observer. For example, schizophrenia patients exhibit differing intraepithelial capillary networks, and when compared to healthy brains, divergent electroencephalogram (EEG) alpha band power signals were first recognized using machine learning algorithms.
Wearable devices can monitor EEG alpha band power signals, usually consisting of a cap or, more recently, a discreet band, assisting in schizophrenia diagnosis. Aside from diagnosing specific conditions relating to the brain, continuous analysis of brain state and function will reveal various tendencies relating to behavior, performance, and probably most aspects of human personality and thought processes. Like the field of genomics, the huge data sets involved will require the use of machine learning to correlate the EEG properties of the brain with other physiological factors, environmental conditions, personal and social events, and so on, ultimately generating a predictive model able to correlate brain states with thought and action.
Benign and simple applications of such technology include monitoring how weary those in critical positions, such as surgeons or flight controllers, are, massively reducing the occurrence of human error due to fatigue. More worryingly, this technology could predict and pre-suppose one's political leanings, propensity towards crime, or ideological standing, thereby impinging on their human rights by imposing segregation or restricting the right to a fair trial.
References:
- Chan, S.-H., Lee, S.-Y., Fang, Q., & Ma, H. (2016). Integration of Bioelectronics and Bioinformatics: Future Direction of Bioengineering Research. Journal of Medical and Biological Engineering, 36(6), 751–754. https://doi.org/10.1007/s40846-016-0185-1
- Mathieson, K., Loudin, J., Goetz, G., Huie, P., Wang, L., Kamins, T. I., Galambos, L., Smith, R., Harris, J. S., Sher, A., & Palanker, D. (2012). Photovoltaic retinal prosthesis with high pixel density. Nature Photonics, 6(6), 391–397. https://doi.org/10.1038/nphoton.2012.104
- Yoo, E.-H., & Lee, S.-Y. (2010). Glucose Biosensors: An Overview of Use in Clinical Practice. Sensors, 10(5), 4558–4576. https://doi.org/10.3390/s100504558
- Casson, A. J. (2019). Wearable EEG and beyond. Biomedical Engineering Letters, 9(1), 53–71. https://doi.org/10.1007/s13534-018-00093-6