According to the World Health Organization (WHO), the rise of antibiotic resistance is one of the world’s most significant threats to public health, food security, and development. While antibiotic resistance can occur naturally, it is being exacerbated by the widespread misuse of antibiotics worldwide.
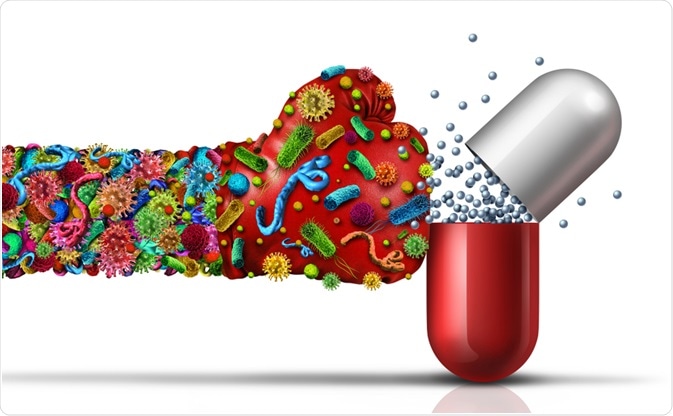
Image Credit: Lightspring/Shutterstock.com
Antibiotic Resistance Explained
As a result of improper antibiotic use, infections that were once easily treatable are becoming harder to manage as antibiotics lose their effectiveness. Diseases such as gonorrhea, pneumonia, salmonellosis, and tuberculosis are becoming increasingly difficult to treat due to rising antibiotic resistance. This growing resistance is placing pressure on healthcare systems, leading to longer hospital stays and increased mortality rates from hard-to-treat infections.
To combat this, understanding how antibiotic resistance develops is crucial. Studies investigating the mechanisms of resistance at the cellular level are key to revealing how bacterial structures like the cell wall contribute to resistance. This article explores current knowledge in this field.
The Structure of Bacterial Cells
Infections are caused by either Gram-positive or Gram-negative bacteria. Gram-positive bacteria have a rigid cell wall surrounding a cytoplasmic membrane, while Gram-negative bacteria have an outer lipid membrane (OM) that encases a thinner cell wall. The space between the OM and the cytoplasmic membrane, known as the periplasm, provides additional protection to Gram-negative bacteria by shielding them from harmful substances.
For decades, scientists have developed antibiotics that compromise bacterial cell walls, causing bacterial lysis. These antibiotics were effective for many years, but due to the increasing misuse of these drugs, bacteria causing serious infections are now becoming resistant.
How Bacterial Cell Structures Contribute to Antibiotic Resistance
While the OM offers protection to bacteria, it also provides opportunities for therapeutic intervention. This membrane contains porins—channels that allow molecules, including antibiotics, to pass through. Scientists have developed therapeutic molecules that exploit these natural structures.
Drug molecules, like antibiotics, can enter bacteria either through diffusion or by utilizing these porins. Hydrophilic molecules pass through the OM via these channels, but a reduction in porin numbers can increase resistance to certain antibiotics, such as β-lactams.
Another mechanism bacteria use to resist antibiotics involves efflux pumps, which are proteins that actively remove antibiotic molecules from the cell, maintaining low intracellular concentrations. Efflux pumps are located in the cytoplasmic membrane and are often multidrug transporters capable of expelling a wide range of antibiotics. As a result, resistance can develop against various antibiotic classes through the action of these pumps.
Other Mechanisms of Antibiotic Resistance
Modification of antibiotic target sites is another common resistance mechanism. Natural variations or mutations in these target sites can prevent antibiotics from binding effectively, reducing their efficacy. Even minor alterations can significantly impact drug effectiveness due to the precision with which antibiotics are engineered to target specific sites.
Several common mutations can lead to antibiotic resistance:
- Alterations in the 30S or 50S subunits of ribosomes result in resistance to antibiotics targeting protein synthesis, including aminoglycosides, chloramphenicol, macrolides, and tetracyclines.
- In Gram-positive bacteria, resistance often arises from modifications to penicillin-binding proteins. For example, Enterococcus faecium becomes resistant to ampicillin through this mechanism. Streptococcus pneumoniae develops resistance to oxacillin and methicillin via a similar pathway. Studies show that the integration of the "staphylococcal cassette chromosome mec" (SCCmec) into the S. aureus chromosome confers resistance.
- Some strains of Enterococcus faecium and Enterococcus faecalis have developed high levels of resistance to teicoplanin and vancomycin by altering their cell wall precursors.
- Resistance to fluoroquinolones can occur due to modifications of the DNA gyrase and topoisomerase IV enzymes, preventing DNA replication and blocking the antibiotic's binding ability.
- Mutations in RNA polymerase can lead to resistance to rifampicin.
Overall, a wide range of genetic mutations can alter bacterial cell structures, resulting in antibiotic resistance. Different antibiotics are threatened by various mutations. Understanding these cellular changes is essential to developing more effective antibiotics that can overcome resistance and continue to treat infectious diseases.
Sources:
- Antibiotic resistance. The World Health Organization. Available at: https://www.who.int/news-room/fact-sheets/detail/antibiotic-resistance
- Kapoor, G., Saigal, S., and Elongavan, A., 2017. Action and resistance mechanisms of antibiotics: A guide for clinicians. Journal of Anaesthesiology Clinical Pharmacology, 33(3), p.300. https://www.ncbi.nlm.nih.gov/pmc/articles/PMC5672523/
- Nikolaidis, I., Favini-Stabile, S. and Dessen, A., 2014. Resistance to antibiotics targeted to the bacterial cell wall. Protein Science, 23(3), pp.243-259. https://www.ncbi.nlm.nih.gov/pmc/articles/PMC3945833/
Further Reading