Chirality relates to the study of the three-dimensional structure of molecules. The term comes from the Greek root kheir-, hand, and means "handedness."
Chiral molecules show left and right-handed nature in the sense that the molecules may exist as mirror-image forms that cannot be made to overlap by any amount of rotation.
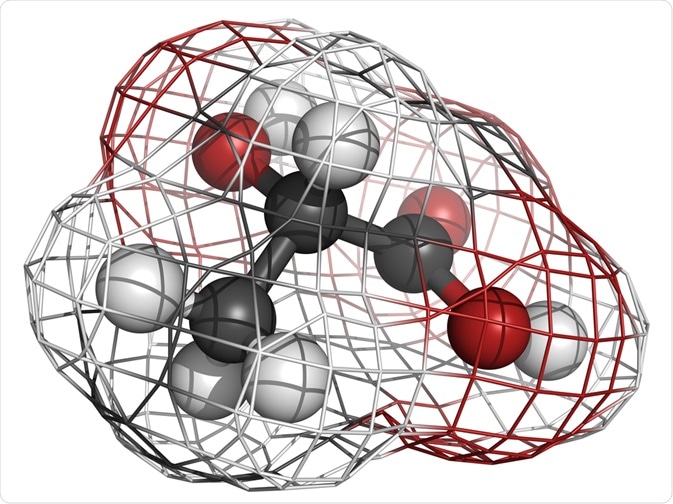
Image Credit: StudioMolekuul/Shutterstock.com
The main cause of chirality in a molecule is that it has an atom (often a carbon atom) that is connected to four different groups in such a way that it is possible to have a non-superimposable image of the molecule.
Such an atom is called a chiral center. Although the carbon atom is the most common chiral center, Si, N, and P atoms are also known to act as chiral centers.
Properties of chiral compounds
The two mirror-image forms of a chiral compound are called enantiomers or optical isomers. Molecules showing chirality have a special property: they rotate the plane of polarized light, and the degree of rotation is called specific rotation or optical rotation.
Those that rotate the plane of polarized light to the right are called (+) or dextrorotatory forms; those that rotate to the left are called (-) or laevorotatory. For this reason, chiral centers are also called optical centers.
Racemic mixtures are those that have both enantiomers in equal proportion. They do not rotate polarized light, as the enantiomeric effects cancel each other out.
When a molecule has more than one optical center, it may have 4 or 6 possible stereoisomers, not all of which may be enantiomers. Such stereoisomers that are not enantiomers are called diastereomers.
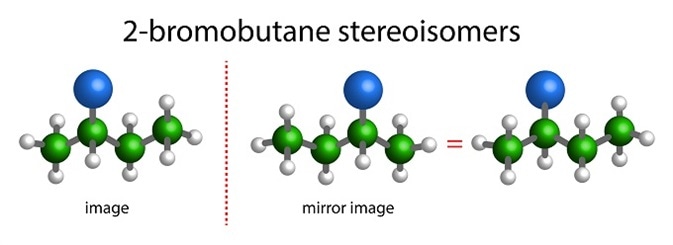
Image Credit: magnetix/Shutterstock.com
Chirality in biomolecules
Generally, when chemical reactions are carried out in solution, there are an equal amount of both (+) and (-) forms in the solution.
A majority of biomolecules (amino acids, proteins, sugars, nucleic acids) are chiral. And in nature, they exist in only one of two possible enantiomeric forms. For example, (-) amino acids are predominant in living systems; (+) forms, on the other hand, occur only rarely (e.g., in antibiotic peptides).
In contrast, most monosaccharides exist only in dextrorotatory forms. Also, an amino acid such as threonine has two optical centers and four enantiomers, but of the latter, only one form is commonly found in nature. All these indicate a preference for one form among all forms of a given molecule.
The most obvious impact of chirality is seen in the shape of molecules. The laevorotatory amino acids always give rise to right-handed helical proteins. Dextrorotatory amino acids, on the other hand, always give the right to left-handed proteins.
A mixture of both forms cannot form helices at all. Similarly, the genetic material deoxyribonucleic acid is always coiled as a right-handed helix.
Functional consequences of chirality in biomolecules
A more important consequence of chirality is that it influences the functional properties of biomolecules in a tremendous way. Simple sugar (glucose) is right-handed and is metabolized by the body. Left-handed glucose, on the other hand, is not metabolized by the body.
The reason is that the enzymes acting on sugar are also chiral and can act only on right-handed sugar. Similarly, proteases can only break down the peptide bonds found in peptides formed with laevorotatory amino acids.
It is known that two enantiomers of a molecule may contribute to different tastes and smells. For example, we know orange and lemon have different scents. The difference in scent is due to the presence of the (+) and (-) forms of limonene present in oranges and lemon respectively.
Similarly, the difference in smell between Caraway seed oil and Spearmint oil is due to the presence of (+) and (-) forms of carvone in these two oils respectively. These imply that the biochemical interactions of the two forms in the nose are different.
The absorption, metabolism, and excretion of enantiomers have also been found to be quite different in animal and human studies. The human body is so selective in its responses to chiral compounds that one isomer may produce the desired effect, while the other may be ineffective, and sometimes even toxic.
For example, one enantiomer of the drug thalidomide is nontoxic while another is known to have dreadful effects on embryonic development.
Chiral separation
Chiral separation of enantiomers is important in the pharmaceutical and agrochemical industries due to the difference in the effects of right- and left-handed enantiomers. Other reasons for separating enantiomers include lower dosing requirements, noninterference by the undesirable enantiomer, as well as cost and time savings.
Further Reading