Artificial chromosomes are not just a synthetic form of the real thing, but a valuable tool for research and potentially ground-breaking therapeutic applications.
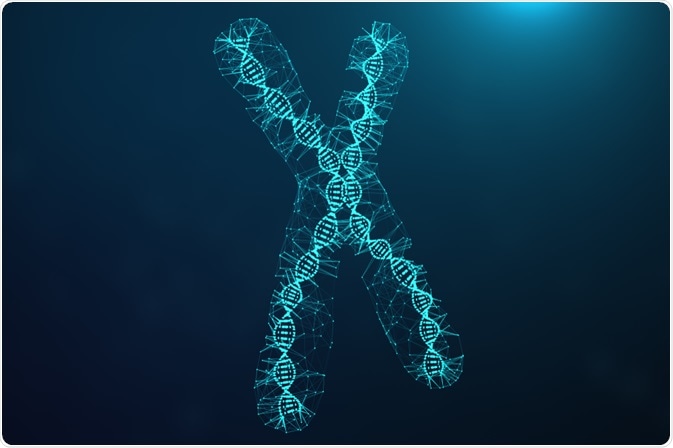
Image Credit: Ustyna Shevchuk/Shutterstock.com
These are scaled-down versions of actual chromosomes that can replicate themselves in the host cells, just like natural chromosomes do, in a stable but non-integrating manner, to introduce large genes or multiple genes into the mammalian or plant cell.
Artificial chromosomes – the need
Structurally, artificial chromosomes are DNA molecules of known composition, synthesized by assembling known components to create a chromosome that acts like a natural one.
The chief advantage of an artificial chromosome is where researchers need to accomplish a much larger piece of gene editing, such as when a piece of DNA over 100 kb—encoding a whole biosynthetic pathway containing numerous enzymes—needs to be added to a chromosome, for instance.
The powerful gene-editing tool CRISPR-Cas9, in contrast, is useful for making precise and specific changes to the genome, but it can’t handle the insertion of complete segments of DNA.
This is where artificial chromosomes prove their worth. They are big enough to hold as much DNA as required, even the huge dystrophin gene with 2.4 million nucleotides.
The first artificial chromosome
In 2014, geneticists first began to build an entire customized yeast chromosome from the budding yeast Saccharomyces cerevisiae.
They assembled a circular plasmid with a yeast centromere, a replication origin, a marker gene, and two stretches of palindromic telomere DNA, using recombinant DNA techniques and inserted it into the yeast, where it became a simple linear molecule.
These plasmids then replicated and segregated with each cell mitosis cycle with over 99% accuracy, and were retained in dividing cultures for at least 20 generations.
By understanding how genes within a chromosome work together to produce the essentials of life and normal metabolism, researchers hoped that they could build plant and animal chromosomes, and even human artificial chromosomes.
However, it is much more difficult to build mammalian artificial chromosomes, for the same reasons that it was easier to build yeast artificial chromosomes.
These reasons include:
- Each of the 3 sequences for yeast chromosome function was well characterized
- Efficient transformation and rapid growth of yeast permit speed, ease, and control
- Yeast have small-sized chromosomes that are easier to assemble
The synthetic chromosome
With increasing knowledge, there are now several ways to create synthetic chromosomes, all based on rebuilding the functional mammalian centromere and telomere while allowing downstream modifications as desired:
1. Defined elements (telomeres, replication origins, and centromeres) are transfected together into a cell line for assembly into an artificial chromosome
2. Individual host cell chromosomes are broken down to the level of minichromosomes, with only essential functional centromere material, by precise removal and insertion of telomeres
3. Targeted amplification of peri-centromere sequences followed by fragmentation, to create satellite DNA-amplified chromosomes (SATAC)
One variation of the SATAC approach is the ACE synthetic chromosome, composed of 50 or more copies of the 245 base pairs bacteriophage lambda attP recombination acceptor sequence, which can be transfected into multiple mammalian cell lines and maintained for long periods, allowing it to be generated within these cells and be readily purified. The system also contains ACE targeting vectors and the ACE integrase.
The centromere obstacle
The greatest hurdle scientists face in this area is the complexity of creating centromeres, the region of the chromosome that is fundamental to the process of replication and cell division.
The defining characteristic of a centromere is the basic protein called histone CENP-A, which is essential for connecting to mitotic spindles.
According to Harvard scientist Alina Chan, the problem is that “centromeres remain poorly defined. We do not know the size requirements, genetic sequence requirements remain ambiguous, and we know only a few proteins that can help to establish a de novo centromere.”
Different approaches have been taken to tackle this problem.
In one method, researchers build up the ‘props’, so to say, to simulate this process. Centromeres are surrounded by repetitive sequences of α-satellite DNA (in humans), which often have CENP-B binding sites (CENP-B promotes the loading of CENP-A on the new artificial chromosome).
Therefore, scientists include α-satellite DNA and CENP-B binding sites in the cloned DNA that they want to convert into a human artificial chromosome. However, this is an inefficient process and often no centromeric DNA is found to be present in the final product.
Another method is preloading yeast artificial chromosomes with over 100 kb of α-satellite DNA from the centromere of chromosome 21 with telomeric DNA and marker genes from the human genome, before introducing them into human cells. Here again, the DNA assembled into stable mini-chromosomes that had no host sequences.
Technical issues
These techniques have problems: firstly, the new chromosomes bear no predictable correlation to the inserted DNA. Secondly, the new rearrangements may not form new chromosomes; instead, this could cause telomeres to seed into the host chromosome, acting as mutagens.
Thirdly, very few successful mini-chromosomes are formed and only within a single type of human cell, the HT1080 fibrosarcoma cells.
Other solutions
Among the new processes being studied to overcome this roadblock, one promising approach is the recruitment of CENP-A into the cloned DNA utilizing a fusion protein. The first step is to incorporate a repeating fragment of DNA called LacO, with a 27-base-pair sequence, into the part of the DNA molecule where the centromere is required to form.
This DNA is then inserted into human cells that have been made to express the fusion protein, containing, among other things, a binding site for LacO and the HJURP protein that puts CENP-A into the chromatin.
The fusion protein binds to the LacO sequences on the introduced DNA via its LacO binding domain, while simultaneously recruiting CENP-A into the chromatin sequences in and near that part of the artificial chromosome via the HJURP protein.
This CENP-A is now ready to form a centromere, and thus converts the cloned DNA into a stable, self-replicating structure that can be called an artificial chromosome.
Other obstacles include making sure large genes are properly expressed and regulated even while inserting them into a human artificial chromosome that must replicate and segregate each time the cell divides.
The transfer process itself is a difficult one and researchers are making improvements to it, as well as to the human artificial chromosomes itself.
Sources
- Greene, A., Pascarelli, K., Broccoli, D., and Perkins, E. Engineering synthetic chromosomes by sequential loading of multiple genomic payloads over 100 kilobase pairs in size. Molecular Therapy Methods and Clinical Development2019, volume 13, P463-473. www.cell.com/.../S2329-0501(19)30041-5
- Logsdon, G. A. et al. Human Artificial Chromosomes that Bypass Centromeric DNA Cell. 2019 Jul 25;178(3):624-639.e19. doi: 10.1016/j.cell.2019.06.006. 10.1016/j.cell.2019.06.006
- Dance, A. (2017). Core Concept: Human artificial chromosomes offer insights, therapeutic possibilities, and challenges. PNAS September 12, 2017 114 (37) 9752-9754; https://doi.org/10.1073/pnas.1713319114. https://www.pnas.org/content/114/37/9752
Further Reading