Though time itself is linear, technology as a whole has undergone advancements on an exponential scale since the industrial revolution. Biotechnology in particular has undergone several paradigm shifts in the fields of medicine, combating the adverse effects on the environment, and human modification.
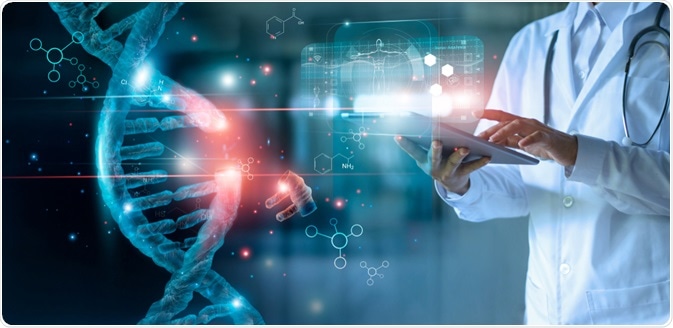
Image Credit: PopTika/Shutterstock.com
To list them all would be a fool’s errand, therefore, this article will be geared towards those advancements that have amassed greater recognition, and promising results for the betterment of humanity. We will expound upon biochemical adaptations on a smaller scale (gene editing) as well as those on a larger scale (tissue engineering).
Endogenous Genetic Manipulation via CRISPR
Corrections for genetic diseases such as cystic fibrosis and muscular dystrophy have been out of reach, until now. While all former genetic manipulation has been done ex vivo, (outside the host’s genome), clustered regularly interspaced short palindromic repeats (CRISPR)’s modus operandi involves editing in vivo through a process analogous to traditional site-directed mutagenesis.
To do this, biological tools are used to construct a segment of DNA, which will then be implemented within a plasmid, and processed in vivo, where the cell can undergo proliferation and a symbiotic relationship with the host.
The CRISPR locus consists of varying segments (shown in the infographic below). First, we have tracrRNA, whose function is to direct the locus to a complementary sequence of crRNA, forming a functional guide RNA (gRNA).
Later, we have varying preinstalled Cas enzymes, the most pivotal of them being Cas9, which performs a cut so that the wild-type DNA and synthetic DNA are joined. These Cas proteins function as RNA-guided endonucleases and perform complex formation with sequence-specific gRNAs. This will form the Cas-gRNA complex, commonly referred to as the ribonucleoprotein (RNP).
Lastly, the direct repeats on the far left of the schematic are used to align the locus in duplex DNA, forming hairpins which will cause preferential formations of apropos Watson-Crick base pairs, maintaining a correct spatial conformation for Cas9 binding.
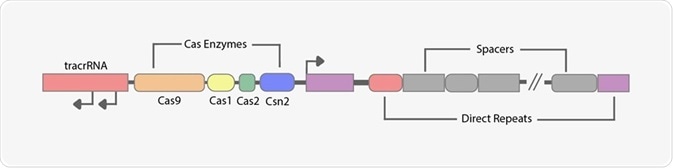
Elementary drawing of Streptococcus pyogenes SF370 CRISPR locus 1-Image Credit: Amalia Medeiros
For this gRNA to recognize the host plasmid and perform a cut, it must first be a specified number of bases away from a protospacer adjacent motif (PAM) sequence located upstream.
Once this cut has occurred, the modified donor DNA can then be implemented through either end-to-end joining, or homologous recombination, achieving the mutation of choice. Most recently, researchers in China have applied this CRISPR technology to treat HIV-1 and acute lymphocytic leukemia, ablating C-C chemokine receptor type 5 (CCR5) in Stem/Progenitor Cells hSPRC’s the first clinical trial relating CRISPR to HIV treatment.
The Manufacturing of Biomaterials for Tissue Engineering
A great many individuals are on transplant lists, and the number of donor organs to sustain the hundreds of thousands of procedures done each year falls short. A prevailing solution to this problem can be found in bioprinting, producing conducting polymers (e.g. PPY, polythiophene) that are compatible with animal models.
Though printing complex internal organs are still within its primitive stages, blood vessels, skin grafts, and other manageable tissues are within reach. Using these polymers in conjunction with tissue printing techniques such as Sacrificial Writing into Functional Tissue (SWIFT), Drop-Based Bioprinting, and Stereolithographic 3D Bioprinting, we can encourage cell integration within a complex biochemical environment.
Within the printing process, artificial tissues such as poly(lactic-co-glycolic acid) (PLGA), and polycaprolactone (PCL) are first immersed in buffers such as polystyrene sulfonic acid and pyrrole DI water mixtures. Then, these conducting polymers emerge from a nozzle attached to the print head, anywhere between 1.0 ± 0.4 cm, and 400 ± 4 μm thick depending on the tissue. Computerized imaging will guide these tissues onto flat surfaces or liquid baths, generating apropos biochemical structures in a method analogous to that of traditional 3D printing.
The resulting prints will often require post-stabilizing methodologies, like UV light, emulation mixtures, etc. Once this has been accomplished, the synthetic tissue will amalgamate with the host, behaving as though it were organic tissue. Bionic eyes, bladders, and even heart tissue has been synthesized using these methods.
Tissue properties and morphologies are extremely complex and providing nutrients and oxygen within these cells has proven to be a challenging feat. Further research on modulating cell behavior is still required, however, great strides have already been accomplished, and the potential for saving lives via bioprinting is extremely promising.
Cells in synthetic tissue will behave like those in organic tissue. Printed bladders, facial nerve regeneration in rats and tissues. Replicating the complex biochemical environment of an organ is extremely challenging. Supplying nutrients and oxygen in all cells of an organ prove to be the most challenging process in this feat. That’s why the greatest successes in this field have are comprised of structures that are flat or hollow.
The moral and sociological implications of deciding whether or not to commit to acts of genome editing, and artificial tissues are topics that have been highly debated since their conception. Many in society today even regard these topics as taboo and fluid when engaging in debate or discourse. However, the bioethicists and genetic councilors of our world today strive to see these changes implemented ethically, without absconding what makes us human.
Sources:
- Conducting Polymers for Tissue Engineering Baolin Guo and Peter X. Ma Biomacromolecules 2018 19 (6), 1764-1782 DOI: 10.1021/acs.biomac.8b00276
- Diverse Class 2 CRISPR-Cas Effector Proteins for Genome Engineering Applications
- Neena K. Pyzocha and Sidi Chen. ACS Chemical Biology 2018 13 (2), 347-356 DOI: 10.1021/acschembio.7b00800
- Shining Light on CRISPR Gene Editing Lapatrada Taemaitree and Tom Brown. ACS Central Science 2020 6 (5), 616-618 DOI: 10.1021/acscentsci.0c00350
- Yu X, Zhang T, Li Y. 3D Printing and Bioprinting Nerve Conduits for Neural Tissue Engineering. Polymers (Basel). 2020;12(8):1637. Published 2020 Jul 23. doi:10.3390/polym12081637
- PRABHUNE, MEENAKSHI. “Full Stack Genome Engineering.” Synthego, Synthego, www.synthego.com/blog/crispr-cure-diseases#other-diseases-that-crispr-can-cure.
- Ensminger M, Löbrich M. One end to rule them all: Non-homologous end-joining and homologous recombination at DNA double-strand breaks. 2020 Feb 28 Br J Radiol. 2020 Nov 1;93(1115):20191054. doi: 10.1259/bjr.20191054.
Further Reading