What is the difference between single- and double-stranded DNA?
Deoxyribonucleic acid (DNA) contains a sugar-phosphate backbone and nitrogenous bases. It encodes genetic information for the growth, functioning, and reproduction of organisms.
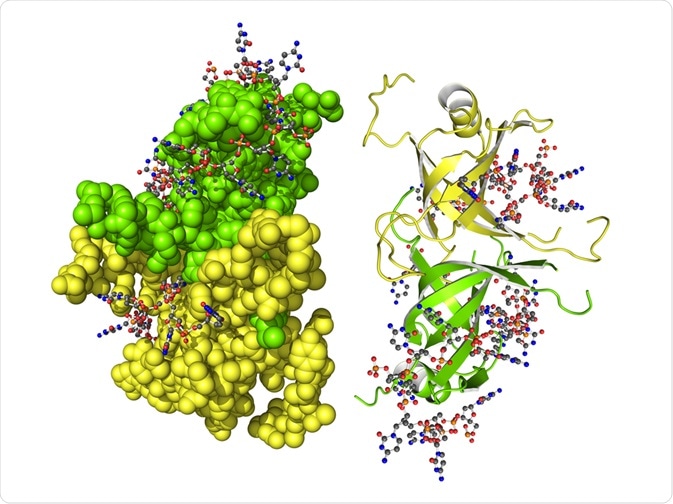
Image Credit: petarg/Shutterstock.com
DNA can exist in different forms – single-stranded (ssDNA) or double-stranded (dsDNA). DsDNA exists in double helixes, where two strands of DNA wind around each other. SsDNA can fold into different shapes but is usually stellate or star-shaped.
The nitrogenous bases can form hydrogen bonds with each other, with adenine (A) paired with thymine (T) and guanine (G) paired with cytosine (C). In dsDNA, due to hydrogen bonding between the base pairs of the two strands, the structure of dsDNA is more stable than ssDNA.
Thus, most organisms use dsDNA to encode genetic information, whereas only a few viruses use ssDNA to store genetic information.
Importance of ssDNA synthesis in nature
All organisms must replicate their DNA when cells divide. During DNA replication, an enzyme called helicase unwinds the dsDNA and opens a replication fork where the two strands of parental DNA are separated.
Single-strand binding proteins bind to the two strands to prevent them from reannealing. Then an enzyme called primase synthesizes a few ribonucleic acid (RNA) bases.
This acts as a primer to allow DNA polymerase to synthesize ssDNA with bases complementary to the bases on the parental ssDNA strand.
Synthesizing ssDNA during DNA replication is important because it allows duplication of genetic material and thus the reproduction of cells.
Another role of ssDNA is to aid with DNA repair. DsDNA can be damaged by external factors such as free radicals and radiation.
Repair mechanism is also required when there are errors during replication such as mismatched base pairs. The base excision repair mechanism is one of the DNA repair mechanisms.
DNA glycosylate recognizes a DNA damage site by abnormal topology when DNA base pairs are mismatched, and an endonuclease removes the strand of damaged DNA, leaving the other ssDNA strand intact.
Then DNA polymerases synthesize ssDNA complementary to the intact ssDNA and the DNA damage is repaired. DNA repair is essential to ensure the correct sequence of the genetic material and prevent mutations that might harm the organism’s well being.
Role of single-stranded DNA in sequencing
DNA sequencing provides a glimpse into the genome and allows identification of genes and mutations. SsDNA plays an important role in DNA sequencing.
In high-throughput sequencing, the sample dsDNA is processed and fragmented. The single-stranded fragments are attached to a glass slide and then base-paired and amplified with a polymerase.
To determine the sequence, reversible terminator bases are added. These are fluorescently labeled nucleotides that terminates the polymerase elongation process once being base paired to the sample ssDNA.
A camera then takes an image and determines the base, A, T, C or G that is newly paired to the sample ssDNA based on the unique fluorescence label of each of the bases.
Reversible terminator bases are reversible because the dye attached to the nucleotide can be removed and the polymerase can incorporate the next base and continue sequencing the whole ssDNA.
Multiple, fragmented sequence reads are then compiled and assembled to elucidate the full sequence of the sample dsDNA.
Also, long-read sequencing utilizes the synthesis of ssDNA. The sample dsDNA is separated into ssDNA and transported through a small well-like container. At the bottom of the well, a polymerase synthesizes ssDNA by incorporating fluorescently labeled nucleotides into the sample ssDNA passing through the well. This allows continuous sequencing and production of long-read sequences without assembly of short sequences.
Role of single-stranded DNA in gene expression analysis
When it comes to analyzing gene expression, the amount of the messenger RNA (mRNA) of a gene reveals the level of gene expression.
The mRNA is quantified using Quantitative reverse transcription Polymerase Chain Reaction (RT-qPCR).
Due to the higher stability of DNA compared to RNA, the mRNA collected is reverse transcribed into complementary DNA (cDNA), which involves the synthesis of single-stranded cDNA with a sequence complementary to the mRNA sequence.
This single-stranded cDNA then acts as a template for PCR amplification. The amount of cDNA can be quantified by back-calculation from the amount of PCR product, and thus quantify the number of mRNA transcripts.
RT-qPCR is an important tool for clinical diagnostics, genotyping, and detection of pathogens.
Role of single-stranded DNA in biotechnology
Synthetic biology is an emerging field that involves engineering organisms for specific purposes in medicine, manufacturing, and agriculture.
De novo synthesis of genetic material is required, when one wants to genetically engineer organisms
One of the most commonly used methods is column-based DNA synthesis. It consists of the addition of nucleotides, one base at a time, to an ssDNA chain attached to a matrix.
An alternative is to use microarray-based DNA synthesis, where the newly synthesized chain of nucleotides is attached to a microchip surface.
To assemble ssDNA fragments into dsDNA, ssDNA fragments are designed to have overlapping extension sequences and are assembled into dsDNA via progressive overlap extension assembly.
Reference:
- Alberts B, Johnson A, Lewis J, et al. Molecular Biology of the Cell. 4th edition. New York: Garland Science; 2002. DNA Replication Mechanisms. Available from: https://www.ncbi.nlm.nih.gov/books/NBK26850/
- Bustin S. (ed) (2004) A-Z of Quantitative PCR. IUL Biotechnology Series, International University Line, La Jolla, California.
- Chatterjee, N., & Walker, G. C. (2017). Mechanisms of DNA damage, repair, and mutagenesis. Environmental and molecular mutagenesis, 58(5), 235–263. https://doi.org/10.1002/em.22087
- Heather, J. M., & Chain, B. (2016). The sequence of sequencers: The history of sequencing DNA. Genomics, 107(1), 1–8. https://doi.org/10.1016/j.ygeno.2015.11.003
- Hughes, R. A., & Ellington, A. D. (2017). Synthetic DNA Synthesis and Assembly: Putting the Synthetic in Synthetic Biology. Cold Spring Harbor perspectives in biology, 9(1), a023812. https://doi.org/10.1101/cshperspect.a023812
Further Reading