Diabetes Mellitus is a metabolic disorder characterized by dysregulation in blood glucose concentrations.
Type 1 Diabetes Mellitus accounts for 5% of cases; it is an auto-immune defined by the destruction of β-cells in the pancreas. Type 2 Diabetes Mellitus (T2DM) is a pleiotropic, metabolic disorder that affects a variety of systems, especially hepatic, skeletal muscle, and adipose tissue. It has significant consequences in terms of cardiovascular disease, cancer, and neuropathies (Xu et al., 2018). Two critical factors are involved in the pathogenesis of T2DM: β-cell physiology dysregulation and insulin resistance.
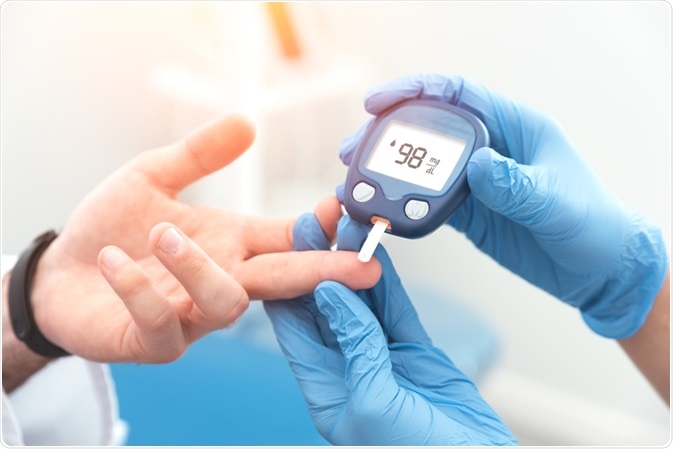
Diabetes. Image Credit: Proxima Studio/Shutterstock.com
Insulin signaling
In healthy individuals, insulin signaling is tightly regulated. A post-prandial spike in blood glucose facilitates its binding to the GLUT2 (Glucose) transporter in the pancreas, specifically on the β-cells in the Islets of Langerhans. The ingress of glucose results in glycolysis, affecting the ATP/ADP intracellular balance.
ATP molecules then bind to the ATP-dependent potassium channels. This, in turn, drives the depolarization of the cell due to the egress of potassium molecules. The depolarization opens voltage-dependent calcium channels, allowing Ca2+ entry. This facilitates the exocytosis of insulin-containing granules.
When insulin binds to its receptor, the insulin receptor undergoes crosslinking and autophosphorylation. This facilitates the Insulin Receptor Substrate (IRS) to bind. This docking protein acts as a nexus for a series of downstream effectors that regulate cell growth, protein synthesis, glucose regulation, and glucose receptors (GLUTs) (Haeusler, McGraw, and Accili, 2018). These processes are often dysregulated in diabetes due to insulin resistance.
β-cell dysfunction in T2DM
The architecture of the Islets of Langerhans is composed of α-cells and β-cells. α-cells produce glucagon while β-cells produce insulin. Paracrine signaling is critical for metabolic homeostasis. Other cells in the region include δ-cells (which produce somatostatin) and ε-cells (which produce ghrelin) (Da Silva Xavier, 2018). Maintaining the integrity of this architecture is critical to physiology (Da Silva Xavier, 2018).
A hyperlipidemic and hyperglycemic diet (often observed in obese/overweight patients) potentiates β-cells to dysregulation. This includes increased cell death due to increased Reactive Oxygen Species (ROS) production from mitochondrial dysfunction. Increased abundance of Free Fatty Acids (FFAs) introduces ER stress, activating the Unfolded Protein Response, accelerating cell death. Hyperglycemia interferes with insulin biosynthesis and can generate islet amyloid polypeptides, driving abnormal insulin generation, increased ROS production, enhancing local inflammation (Halban et al., 2014).
Mitochondrial dysfunction
An emerging factor in diabetes research is mitochondrial dysfunction. Nutrient overload dysregulates both mitochondrial function and turnover. Patients with obesity and insulin resistance exhibit reduced oxidative phosphorylation and impaired lipid metabolism.
Mitochondria are responsible for energy generation through the Electron Transport Chain. This is a tightly regulated process involving a series of complexes; nutrient overload disrupts this delicate arrangement. This results in the production of O2- free radicals. This activates the several major pathways involved in the pathogenesis of diabetes.
In response to increased ROS production, the mitochondria will trigger apoptosis or mitophagy. These drive the release of factors such as ceramide and Diacyl Glycerol (DAG). DAG interferes with IRS phosphorylation while ceramide inhibits AKT (this regulates the translocation of GLUTs. T2DM dysregulation also affects Peroxisome proliferator-activated receptor (PPAR) and Peroxisome proliferator-activated receptor-gamma Coactivator-1α (PGC-1α): both affect lipid metabolism but also mitophagy biogenesis (specifically mitofusin-2).
If mitophagy and mitogenesis are impacted, this generates a cycle of reduced ATP generation, increased ROS, and impaired metabolism. Thus, promoting an environment that leads to metabolic dysregulation. Certain systems are particularly sensitive to the dysregulation of insulin.
Insulin Resistance in Skeletal Muscle
Under basal conditions GLUT1 passively uptakes glucose. GLUT4 is responsible for insulin-mediated glucose uptake. Research indicates that a diabetic phenotype affects the translocation of GLUT4 receptors rather than expression. Insulin stimulates glycogen synthesis in skeletal muscle by driving glucose uptake.
Physical activity and weight loss remain the cornerstone for the treatment of T2DM. Its phenotype has a pronounced effect on skeletal muscle. Low-level inflammation is a marker for diabetes: exercise regulates the generation of anti-inflammatory factors such as IL-1 receptor antagonist and soluble TNF receptor.
Exercise also induces the generation of antioxidants such as glutathione which dampen the oxidative stress associated with the diabetic phenotype. PGC-1α plays a critical, multi-faceted role in metabolism, including glucose homeostasis, mitochondrial biogenesis, and lipid catabolism. Exercise acts through PGC-1α to manage blood glucose (Summermatter et al., 2013). Irisin, a myokine, regulates glucose tolerance in muscle and adipose during exercise.
Insulin Resistance and the Liver
The liver is one of the most important sites for metabolic homeostasis as it regulates glucose/glycogen metabolism as well as lipid metabolism. It also generates critical hormones and factors that are often dysregulated in diabetes. Insulin signaling in hepatocytes modulates glucose metabolism through a variety of mechanisms. AKT acts as a sensor and regulates downstream effectors. FOXO regulates gluconeogenesis. Glycogen synthase regulates glycogen synthesis. GLUT4 translocation is also governed by AKT.
Insulin resistance fails to regulate these processes, exacerbating a hyperglycemic and hyperlipidemic environment by impairing glycogen storage and lipogenesis. It also inhibits the ability of insulin to inhibit hormone-sensitive lipase, further accelerating the lipid-rich environment. The abundance of FFAs enhances gluconeogenesis. Nutrient overload desensitizes the liver to insulin, preventing it from regulating the homeostasis of carbohydrate and lipid metabolism, promoting a pre-diabetic phenotype.
Insulin Resistance and Adipose Tissue
Adipocytes stimulate either the uptake of glucose and triacylglyceride production or suppressing its hydrolysis and promoting the uptake of FFAs and glycerol to store fat. This depends on nutrient abundance. Insulin resistance can be observed in adipocytes. This is very pertinent in hypertrophic adipocytes. Dysregulated AKT impairs GLUT4 translocation and drives lipolytic enzymes exacerbating hyperglycemia.
FFAs from adipocytes can migrate to hepatocytes and skeletal muscle, worsening metabolic impairment. A hyperlipidemic environment triggers adenine nucleotide translocase 2. This induces hypoxia and induction of Hypoxia Inducible Factor (HIF-1). HIF-1 regulates pro-inflammatory factors and contributes to the low-level inflammatory environment in diabetes. Adipokines such as adiponectin can modulate the diabetic phenotype.
Adiponectin inhibits β-cell death by eliminating toxic factors such as ceramide and DAG. It modulates liver and muscle fatty acid oxidation, improves glucose uptake in muscle and white adipose, and mitigates inflammation in adipose. Adiponectin is downregulated in patients with diabetes (Galicia-Garcia et al., 2020).
Sources:
- Galicia-Garcia, U. et al. (2020) ‘Pathophysiology of Type 2 Diabetes Mellitus’, International journal of molecular sciences, 21(17), p. 6275. doi: 10.3390/ijms21176275.
- Haeusler, R. A., McGraw, T. E. and Accili, D. (2018) ‘Biochemical and cellular properties of insulin receptor signalling’, Nature Reviews Molecular Cell Biology, 19(1), pp. 31–44. doi: 10.1038/nrm.2017.89.
- Halban, P. A. et al. (2014) ‘β-cell failure in type 2 diabetes: postulated mechanisms and prospects for prevention and treatment.’, Diabetes care, 37(6), pp. 1751–1758. doi: 10.2337/dc14-0396.
- Da Silva Xavier, G. (2018) ‘The Cells of the Islets of Langerhans’, Journal of clinical medicine, 7(3), p. 54. doi: 10.3390/jcm7030054.
- Summermatter, S. et al. (2013) ‘PGC-1α Improves Glucose Homeostasis in Skeletal Muscle in an Activity-Dependent Manner’, Diabetes, 62(1), pp. 85 LP – 95. doi: 10.2337/db12-0291.
- Xu, G. et al. (2018) ‘Prevalence of diagnosed type 1 and type 2 diabetes among US adults in 2016 and 2017: population based study’, BMJ, 362, p. k1497. doi: 10.1136/bmj.k1497.
Further Reading