Most medications are still delivered by oral administration even today. However, when it comes to lung diseases, inhaled drugs are at the forefront of modern management, whether it is asthma, chronic obstructive pulmonary disease, cystic fibrosis or pulmonary hypertension.
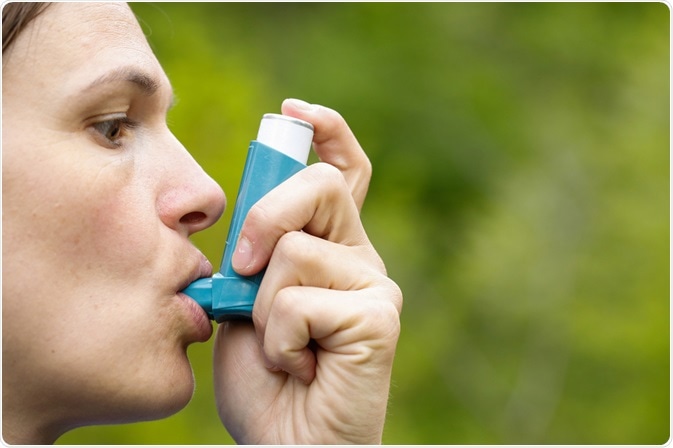
Image Credit: zlikovec/Shutterstock.com
Pulmonary drug delivery is an attractive option for many other therapies too, such as lung tuberculosis, chronic lung infections, and type 2 diabetes mellitus.
The advantages of inhalation
Inhaled drugs reach the site of disease directly, making them more effective and reducing their adverse effects as less of the drugs reach the systemic circulation.
There are a variety of formats, such as jet and mesh nebulizers, metered-dose inhalers (MDIs), and dry powder inhalers (DPIs).
Still, more adaptations are coming on the scene, such as breath-actuated, electronically monitored or feedback-actuated technologies, to increase their safety, convenience, and efficacy.
Biopharmaceuticals, like recombinant human insulin, could also be given by inhalation, avoiding the need for injection. Studies show that this is a more efficacious and rapid-acting route. Initially, scientists imagined creating a plethora of potential inhalable drugs, such as hormones, antidepressants, interferons, and opioids.
The challenges of inhalable drugs
Despite the obvious advantages, inhalable formulations are a challenge: they must be stable at room temperature, efficiently delivered in a reproducible dose and simple to use.
Formulation issues include drug absorption, particle size, models for toxicology parameters and patient compliance. Several factors are affecting the deposition of drug particles in the respiratory tract, such as inertia, gravitational sedimentation, and Brownian motion.
Drug absorption
Lungs have an enormous surface area, making them ideal for drug absorption. They are composed of two different parts, the cartilage-lined tracheobronchial tree and the thin-walled alveolar sacs lined by a dense capillary network, making up 95% of the whole.
However, therapeutics delivered to the lungs to treat lung conditions need to remain within the lungs rather than be absorbed, since they could have significant adverse effects on the gut, the heart, the brain, and the blood vessels.
This requires a reversal of traditional formulation routes which are aimed at improving absorption and bioavailability.
Drug particle size
Drugs delivered as aerosols through MDIs or DPIs must have the right range of particle size. This is because this parameter is crucial to the deposition of the appropriate dose, as well as the drug’s distribution to the right location.
If the particles are large (>10 μm), the aerosol will land on the oropharynx and larynx due to inertia, While if the size is between 2–5 μm, the particles land in the central airways.
Small particles (0.5–2 μm) will reach the peripheral airways. If too small (below 0.5 μm), they drift about without settling and leave the lungs with the next breath.
Another issue with very small particles is the danger that they may adhere to form larger than intended particles, irritating the airways as well as reducing the efficacy.
Drug concentrations vary with the location where the particles land, as well as with the occurrence of receptors in that site.
Nanoparticles – magic bullets?
When it comes to nanoparticles (NP), however, they agglomerate and thus reach the alveolar region in pharmacologically useful doses by sedimentation. This keeps them in the respiratory tract for a longer time and boosts biological activity.
Other advantages include their sustained release, evasion of phagocytic activity, resistance to chemical or enzymatic breakdown and specificity of attachment, as a result of decoration with molecules that attach the nanoparticle to the mucosa or bacterial biofilms.
On the other hand, the small size of NPs makes them liable to be expelled during exhalation. This has been addressed by either using them in colloidal form or by embedding them with micron-sized carriers.
Another hindrance is the lack of inhalable excipients, which limits the repertoire to mannitol, lactose, leucine, and dipalmitoylphosphatidylcholine (DPPC)—a phospholipid found in lung surfactant. Stable NPs in powder form is achieved using methods like lyophilization or spray-drying.
The micronization methods used to prepare nanoparticles of a suitable size for delivery to the lungs may create particles with irregular surfaces, causing the drug to reform crystals during its storage. This could make the drug less effective and, therefore, post-micronization of the particles must often be treated.
Toxicity modeling
However, detailed toxicity tests need to be done in vitro and in vivo, to ensure that inhaling NPs, even those generally recognized as safe (GRAS), will not be a toxic process. The polymers used for the NPs must be compatible with lung fluid to avoid accumulation in the peripheral lung, where mucociliary clearance is absent. This can deplete surfactant, attract phagocytes and cause inflammation.
Another issue may arise if the product contains a residual solvent, which is toxic to lung tissue. Hypersensitivity to sugar or cyclodextrin carriers may cause bronchoconstriction in susceptible individuals. Finally, carriers, enzyme inhibitors, and other molecules may elicit an immune or irritant reaction, with increased permeability allowing toxins and antigens to pass across the epithelial barrier.
The complex processing of NPs could be a hindrance to the commercial production of these drug formulations. Eventually, there is often a surprising disparity in aerosolized drug bioavailability between animals and humans. For instance, growth hormone is absorbed to the tune of 45% in animals but only 3%–10% in humans. Large-molecular-weight antibodies are not absorbed unless a transporter is used.
Conclusion
Researchers suggest that the rapid absorption and effects produced by inhaled drugs are persuasive reasons to favor their use, rather than oral or injectable drugs, in conditions such as nicotine addiction, migraines, Parkinson’s disease, and insomnia. This could improve the potential control and quality of life.
On the other hand, time and patience are required to convince the public and the professional healthcare providers about the benefits of this new approach, which has been shown to outperform oral drugs in several areas by reducing the dose required—and thereby the toxicity—while maintaining the efficacy and improving compliance.
Sources
- Cipolla, D. Will pulmonary drug delivery for a systemic application ever fulfill its rich promise? Expert Opinion on Drug Delivery 2016, Volume 13, Issue 10, Pages 1337-1340. https://doi.org/10.1080/17425247.2016.1218466. https://www.tandfonline.com/doi/full/10.1080/17425247.2016.1218466
- Russell, V. Four Challenges for Pulmonary Drug Delivery. Pharmaceutical Technology Volume 2017 Supplement, Issue 4, s16-s18. https://www.pharmtech.com/four-challenges-pulmonary-drug-delivery
- Moreno-Sastre, M., Pastor, M., Salomon, C., Esquisabel, A., and Pedraz, J. L. Pulmonary drug delivery: a review on nanocarriers for antibacterial chemotherapy. Journal of Antimicrobial Chemotherapy, Volume 70, Issue 11, November 2015, Pages 2945–2955, https://doi.org/10.1093/jac/dkv192. https://academic.oup.com/jac/article/70/11/2945/2363606
- Patil, J. S., and Sarasija, S. Pulmonary drug delivery strategies: A concise, systematic review. Lung India. 2012 Jan-Mar; 29(1): 44–49. DOI: 10.4103/0970-2113.92361. https://www.ncbi.nlm.nih.gov/pmc/articles/PMC3276033/