Among the various spectroscopic techniques in circulation, Raman spectroscopy uses the interaction of light with matter to investigate the vibrational modes of molecules, providing detailed information about the molecular structure of a sample.
Each vibration is influenced by the chemical environment (i.e. nature of the chemical bond, inter- and intermolecular forces) and the respective signal has a characteristic position and intensity. Raman spectroscopy can therefore reveal a sort of structural fingerprint for the identification of molecules.
The technique can be widely used in several fields, it is non-destructive, requires little or no sample preparation, and can be used for both quantitative and qualitative purposes.
Principles of Raman Spectroscopy – the Raman Effect and the spectrum
Raman spectroscopy is based on the phenomenon of inelastic scattering, or Raman Effect (after the scientist who discovered it). When a sample is hit by a monochromatic laser beam — typically in the visible or near-infrared range — the radiation interacts with the vibrational modes of the molecules of the sample and then scatters in all directions.
Most often, the scattered radiation has the same frequency as the incident radiation (Rayleigh scattering). However, a small part of the scattered light has a frequency that differs from that of the incident one (inelastic scattering).
Collisions, where the frequency of the incident radiation is higher than the frequency of the scattered radiation, generate the so-called Stokes lines in the Raman spectrum. Conversely, anti-Stokes lines are observed when the frequency of the scattered light is higher than that of the incident one.
Since Stokes shifts involve transitions from lower to higher energy vibrational levels, Stokes bands are more intense than anti-Stokes bands, and consequently are more easily observed in Raman spectroscopy.
Unlike infrared spectroscopy (IR) that requires the molecules to have a dipole momentum to be IR active, a vibration is Raman active when there is a change in the induced dipolar momentum (or polarizability - α) of the molecule.
Raman is often used as a complementary technique to IR for structural elucidation. Moreover, Raman scattering due to water is low, therefore water is an ideal solvent for dissolving samples (which is instead not possible in conventional IR spectroscopy).
Raman spectra are reported as intensity versus Raman shift (wavelength shift, expressed in cm-1), with most of the vibrations of organic molecules occurring in the range 4000-40 cm-1. In addition to lab-based Raman spectrometers, portable and hand-held devices for in-field or in-situ analysis are also available.
Signal amplification with SERS
There are instances where conventional Raman spectroscopy can suffer from the low intensity of the signals as well as interference from sample fluorescence. The development of Surface-Enhanced Raman Spectroscopy (SERS) has overcome these limitations. SERS permits the study of surface interactions, adsorption processes, and biological systems, just to name a few examples.
At the basis of SERS is the ability of some nanostructured metal surfaces (generally gold and silver), to develop large electromagnetic fields localized in small regions, when illuminated by radiation with appropriate frequency. Sample molecules adsorbed on the surface that enters these regions, generate more Raman scattered photons, producing therefore a much stronger Raman signal.
However, SERS has its limitations when it comes to the analysis of complex samples since certain analytes will be adsorbed more than others. Consequently, their signals in the spectra will dominate over those of the less adsorbed molecules.
Applications of Raman Spectroscopy
Raman spectroscopy can find applications in various sectors, from the industrial analysis of raw materials and monitoring of manufacturing processes to forensic analysis. Moreover, many studies are focussing on macromolecule and biomaterial characterization, particularly regarding polymeric nanoparticles for drug release and drug detection.
Raman was used for the real-time monitoring of drug release by gold nanoparticles, both in vitro and in vivo. Such nanocarriers were made with poly(ethylene glycol) (PEG) spacers containing −SH and −COOH functional groups, and were in turn used for covalent binding of mitoxantrone, an amine-containing anticancer drug.
The study revealed that the drug delivery vehicles accumulated in the heart of healthy mice after 5 min of administration and infiltrated in the lung tumor site of unhealthy mice after 5 h of injection.
SERS could potentially be used for the quality-control method of certain cell culture media components, such as the detection of adulterants like melamine in biopharmaceutical raw materials (urea, sucrose, arginine, and histidine) and commercially available cell culture media.
SERS is effective for investigating molecules containing sulfur and nitrogen since they can efficiently couple with the silver and gold surfaces, and the study showed that melamine could be detected at trace levels in some raw materials in low ppm levels.
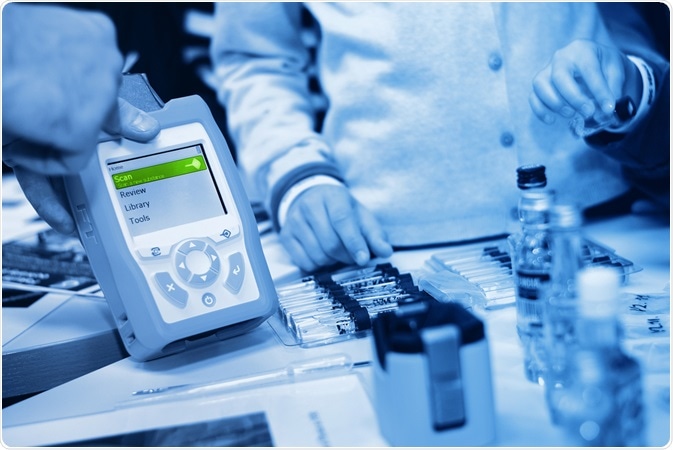
Image Credit: Forance/Shutterstock.com
Conclusion
Although there are some limitations, such as low sensitivity and fluorescence interference, Raman spectroscopy has emerged as a non-destructive technique for quantitative and qualitative analysis in several settings. With new technological developments shortly, it is expected that Raman spectroscopy will become a popular analytical tool for a growing number of applications.
References
- Buckley, K. & Ryder, A. G. (2017). Applications of Raman Spectroscopy in Biopharmaceutical Manufacturing: A Short Review. Appl Spectrosc, 71, 1085-1116.10.1177/0003702817703270
- Bumbrah, G. S. & Sharma, R. M. (2016). Raman spectroscopy – Basic principle, instrumentation, and selected applications for the characterization of drugs of abuse. Egyptian Journal of Forensic Sciences, 6, 209-215.10.1016/j.ejfs.2015.06.001
- Tian, F., Conde, J., Bao, C., Chen, Y., Curtin, J. & Cui, D. (2016). Gold nanostars for efficient in vitro and in vivo real-time SERS detection and drug delivery via plasmonic-tunable Raman/FTIR imaging. Biomaterials, 106, 87-97.10.1016/j.biomaterials.2016.08.014
- Wen, Z. Q., Li, G. & Ren, D. (2011). Detection of trace melamine in raw materials used for protein pharmaceutical manufacturing using surface-enhanced Raman spectroscopy (SERS) with gold nanoparticles. Appl Spectrosc, 65, 514-21.10.1366/10-06089
Further Reading