The 5' untranslated region (5' UTR) is a sequence present in messenger RNA (mRNA), which serves as the point of entry for the ribosome during translation. This primary structure can adopt RNA secondary and tertiary structures that regulate translation initiation in one of two ways.
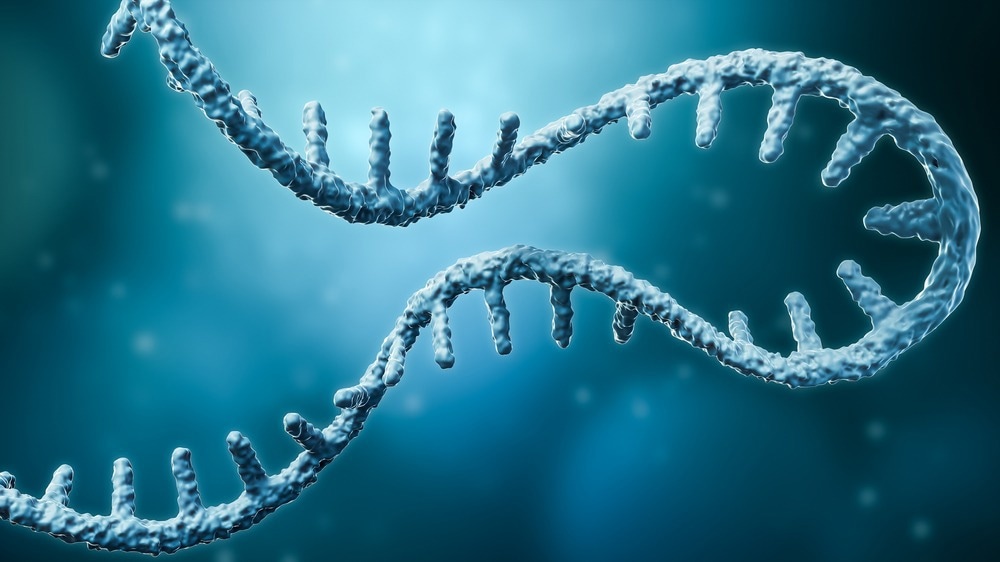
Image Credit: MattLphotography/Shutterstock.com
Complex RNA structures present in 5' UTRs, such as RNA G-quadruplexes, may play the role of a steric block two RNA structure unwinding through scanning the ribosome, eucaryotic initiation factor 4A (elF4A), and helicases.
RNA is hypothesized to have served as the primary catalytic, self-replicating, and information-dense component of cellular life in the ancient world. The ability of these molecules to maintain these characteristics is attributed to RNA folding into complex secondary and tertiary structures. As a consequence, there are several RNA structure-directed functions which include their role as ribozymes and metabolite-sensing riboswitches.
RNA primarily transmits information through its coding sequence. However, it possesses a 3' and 5' UTR, non-coding sequences that do not directly contribute to the construction of a protein during translation.
Consequently, it is not subject to the same traditional (canonical) base-pairing rules, i.e., Watson-Crick, and can instead form several non-canonical base pairing to modulate translation. These based pairing patterns can subsequently respond to the binding of proteins involved in translation and impact how ribosomes are recruited. Algorithms have predicted the prediction of these binding and modulation patterns. The past decade has seen advances in these algorithms as they have been integrated with experimental RNA structure-probing methodologies.
Canonical Cap-Dependent Translation Initiation
RNA secondary and tertiary structures may regulate translation initiation in a cap-dependent or cap-independent manner. In canonical cap-dependent translation initiation, translation is initiated once a ribosome is recruited to the 5' cap, and the ribosome begins scanning towards the start codon. The recruitment of the ribosome begins with the recruitment of the 40S small ribosomal stop unit and its associated eucaryotic initiation factors (eIFs). These components are recruited to the 5' end of the 7-methylguanosine (m7G) cap structure.
To begin with, the trimeric eIF4E Factor interacts with the scaffolding initiation factor eIF4G and the RNA helicase eIF4A. eIF4G interacts with poly-A binding protein (PAPB), which is recruited to the three' poly(A) tail; this subsequently circularises the mRNA. The eIF4G-eIF3 interaction recruits the 43S pre-initiation complex. The 43S pre-initiation complex is composed of the 40S ribosomal subunit, a ternary complex of GTP-bound eIF2 and the initiator tRNA (eIF2–GTP–Met-tRNAi), and eIF1, eIF1A and eIF5.
After, the 43S complex binds the mRNA near the cap and scans the 5' untranslated region (UTR) in the 5' to 3' direction using ATP; there is partial hydrolysis of the eIF2-bound GTP to GDP in the ternary complex, which also powers the movement. Once the start codon (AUG) is identified, RNA helicase, with the 43S complex, unwinds secondary structures in the 5' UTR, which inhibit translation.
Stable binding of the 43S complex at the start codon causes the 48S initiation complex to form. The eIFs are fully released via complete GTP hydrolysis, and the 60S large ribosomal subunit is recruited, now joining the 40S ribosomal subunit. Together, the elongation-competent 80S ribosome is formed, and translation elongation begins.
Suppose the cell is subject to starvation and other stress conditions. In that case, the formation of the ternary complex is blocked via phosphorylation of eIF2α and sequestering of eIF4E to block the assembly of the eIF4F.
5' UTRs as Platforms For RNA Structure
The 5' UTR is between 53 and 218 nucleotides in length. The longest known median length occurs in humans; however, 5' UTRs vary considerably in length among the higher eukaryotes, ranging from single digits to a few thousand base pairs.
The structure of 5' UTR can be predicted using the GC content and the folding free energy level. According to canonical translation initiation, the 43S pre-initiation complex scans along the mRNA to find the start codon; a high GC content is thought to cause inefficient scanning and decrease the initiation rate. This has been demonstrated as GC-rich 5' UTRs are correlated with translation inhibition. Overall, predictions calculate the most stable base pairing of an RNA – this is the one with the lowest calculated folding free energy.
The folding free-energy takes into account the complete unfolding of an RNA domain to a completely linear form; as such, they may overestimate what happens in vivo, as scanning is thought to require only local melting of RNA structures rather than complete linearisation.
Nonetheless, it is difficult to predict the global 5' UTR m RNA structures; however, there are distinct 5' UTR RNA motifs in the effort to regulate mRNA translation.
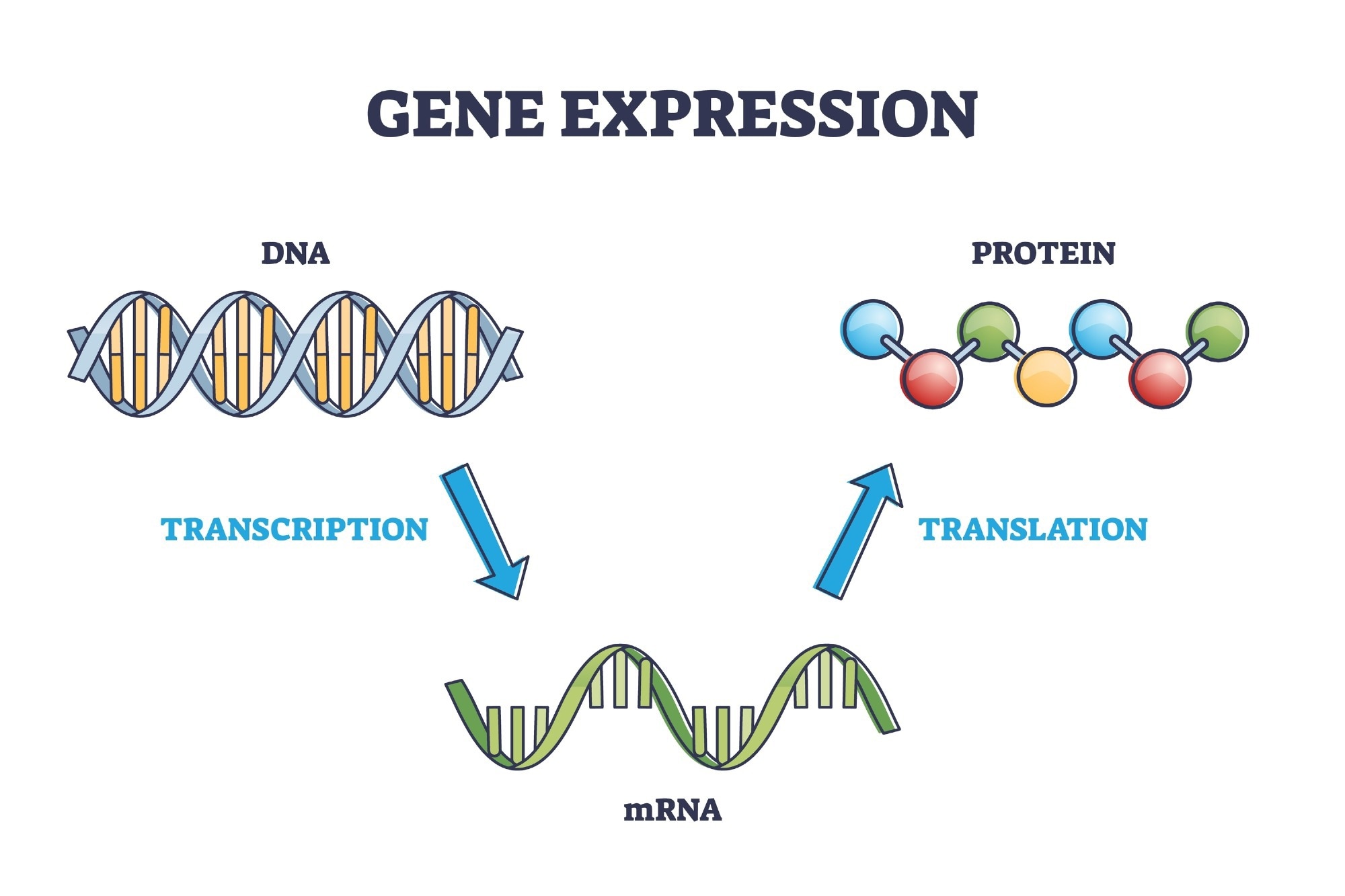
Image Credit: VectorMine/Shutterstock.com
5' UTR Structure and Function
One of the first and best-studied examples is a small 5′ UTR structural element — the iron-responsive element (IRE). This affects the translation of mRNAs involved in iron homeostasis. This structure forms a stem-loop close to the mRNA cap that encodes the iron storage protein ferritin or the iron transporter ferroportin. The latter is bound by iron regulatory protein (IRP1) or IRP2 in low iron conditions. The binding of IRP represses translation initiation. Together, the IRE-IRP-ribonucleoprotein (RNP) complex prevents access by the ribosome to the cap and the 5' UTR.
Although stable RNA secondary structures formed in the 5' UTR include happens. In other instances, 5' UTR RNA may form pseudoknots, hairpins, and other structures called RNA G-quadruplexes (RG4s). These serve to inhibit translation; to overcome this, modifications or the recruitment of RNA-binding proteins and long non-coding RNAs may bind or form RNPs, causing translation initiation.
Studies based on ribosome profiling have been conducted to understand the function of the RNA helicase in translation initiation. To do this, small-molecule inhibitors of the enzyme, such as, have been used. This and other drugs blocked the dissociation of the helicase from the RNA; in these studies, mRNAs that have long 5' UTRs have been shown to produce lower translation efficiency. Many of these transcripts, sensitive to Silvestrol, can encode a specific 12-nucleotide long (CGG)4 motif that can fold into RG4 structures in vitro. This is important in cancer research, as the RNA helicase is hyperactivated in cancer. The folding of (CGG)4 motifs into stable, energetically favorable RG4 structures in vitro have been shown (in ribosome profiling studies) to reduce translation initiation at hundreds of mRNAs. Moreover, the complexity of the inferred structures and the increased length of their 5' UTRs is correlated with the sensitivity to the RNA helicase inhibition.
RNA G-quadruplex Structures
This structure is considered the most stable awareness structure that can block ribosome scanning. In addition, the physiological roles of the structures include roles in mRNA processing and the regulation of translation. RG4s are thought to repress translation, presumably by preventing the formation and binding of the 43s pre-initiation complex or impeding its subsequent scanning activity. However, the inhibitory effect of 5' UTR RG4s on scanning is still speculative.
Higher-Order mRNA Structures
- Pseudoknots are complex intramolecular RNA structures with two or more intercalated stem-loop structures forming three-dimensional knot shapes. This has been proposed to arise in the human interferon-gamma (IFNG) 5' UTR mRNA. In this setting, initiating ribosomes usually unfold the pseudoknot in the IFNG 5' UTR. But it can refold to attract a PKR dimer; this is thought to locally activate the kinase, which phosphorylates eIF2α and represses IFNG translation. This is an example of how this RNA structure, as a positive feedback loop, adjusts the translation of its mRNA to the activity of PKR to prevent the excess synthesis of interferons
- RNAs can form complexes with trans-acting long non-coding RNAs (lncRNAs) for post-transcriptional control
There is a wealth of evidence to suggest that there is a diverse repertoire of mechanisms that translation is controlled by the structures of 5' UTRs. For many of the structures that have been unearthed, however, it is not entirely clear whether or not they serve to improve or hinder the access of the translation machinery. To better find unconfirmed functional mRNA structures in the 5' UTR, more genome analysis is needed using genome alignments to combat conservation and sequence covariation analysis.
New global probing tools are now capable of assessing RNA structures in cells. As such, they may enable the discovery of novel RNA structures in complex genomes as well as analyze their contribution to gene regulation. These will also need to be validated experimentally. As experimental tools continue to improve, they can be applied in the context of development, disease, and cell differentiation - this will prove to be insightful as modes of gene regulation using structure-function relationships in the 5' UTR are highly processed and cell type dependent.
Sources:
- Leppek K, Das R, Barna M. (2018) Functional 5' UTR mRNA structures in eukaryotic translation regulation and how to find them Nat Rev Mol Cell Biol. doi:10.1038/nrm.2017.103.
- Hinnebusch AG, Ivanov IP, Sonenberg N. (2016) Translational control by 5'-untranslated regions of eukaryotic mRNAs. Science. doi:10.1126/science.aad9868.
Further Reading