Analytical chemistry is incorporated in all subsections of chemistry itself; materials science is no exception. Materials science, in its essence, is the study and assay of solid matter and how the property of this solid matter is altered through variations in structure and composition.
A large portion of the experts in this field approach the construction and study of polymeric composites, performing alterations on the molecular level. These finely tuned chemical alterations can relate to temperature, stability, malleability, strength, etc. This article will delve into the current findings of the field and how careful analysis of these materials can lead to advancements in green science and polymers.
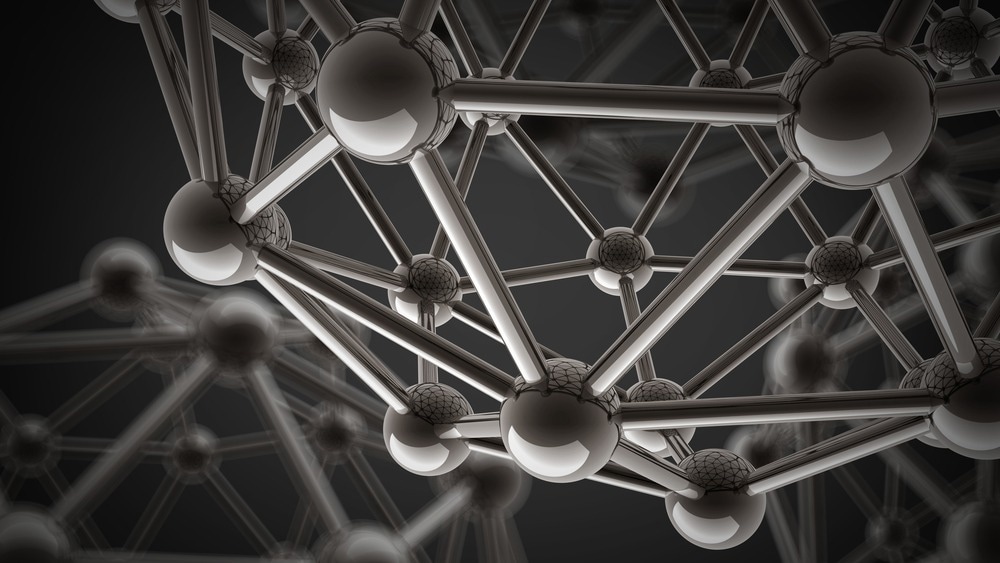
Image Credit: Iaroslav Neliubov/Shutterstock.com
The Interdisciplinary Relationship Between Materials Science and Analytical Chemistry
There exists quite a bit of cross-pollination between materials science and chemistry. The duo shares strong foundations in math and physics, though materials science will incorporate more physics, and practicing either one entails an inherent prowess in the field of kinetics and thermodynamics.
Analytical chemistry, in its essence, is the study of processing and garnering information about the nature of matter. When fitted to materials science, we reduce that scope to garnering information on "solid-state matter".
How Materials Science is Being Used in Recycling
Recycling has been attracting a great amount of media coverage, especially pertaining to how archaic forms of polymer chemistry have deposited a jeopardizing number of plastics within our environment. Therefore, leading researchers are attempting to generate more favorable/ green composites. The unification of materials and green analytical chemistry is not solely limited to recycling, as new materials are being made to filter cleaner air and water, produce renewable "green energy, " and nourish us in ways that do not endanger the planet.
A study by Max J. H. Worthington et al. advocates recycling sulfur into useful polymers. One of the largest byproducts of the petroleum industry is sulfur, which can be converted into elemental sulfur through a process known as "desulfurization". From here, it can create rubber, replenish the stock of sulfuric acid (an essential resource for every country), and generate much-needed fertilizer. Creating these useful monomers from the tens of millions of tons of sulfur created from petroleum refining will drastically decrease our carbon footprint.
Polymer Chemistry
Polymers are extremely prominent in the field of materials chemistry. Today scientists endeavor to explore their potential in radiation resistance, nuclear properties, molecular magnets, and more. These macromolecules (comprised of smaller subunits) are synthesized in the lab to create plastics and graphene for pencils but are also synthesized naturally, such as wool and bodily proteins.
The most common polymer people are familiar with is graphene, which makes up the traditional pencil. Comprised of layers of carbon, the hexagonal arrangement allows the two-dimensional sheets to layer on top of other surfaces.
One variant of polymer that has proven instrumental to our society are polymers of intrinsic microporosity (PIMs). These polymers contain micropores that are roughly 2-3 nanometers in size and harbor a wide variety of applications. Traditionally comprised of two or five-membered rings, these PIMs have a certain rigidity, though we can manipulate the atomic structure to rearrange, factoring in the many bends that comprise the polymer chain. Other polymers differ in this regard, as they have flexible backbones. By altering this backbone, while keeping the dispersity (Đ) to a minimum (roughly 1 unit value), we can create a strong yet contorted polymer chain dissolved in certain solvents.
These polymers' strong, flexible, and dissolvable nature makes it possible to isolate them in a film, powder, coating, or fiber. This is what contributes to their versatility in applications. One of just many examples of how these PIMs can be used is in gas masks. When made into a fluorescent film, these polymers can adsorb organic vapors.
Used predominantly in hazard and construction jobs, this equipment will protect against toxins, carcinogens, and more. The activated carbon that comprises their hull is cheap and readily absorbs. An additional benefit is that the film will alter in fluorescence once it has reached its absorption limit, indicating to workers when it is appropriate to change masks.
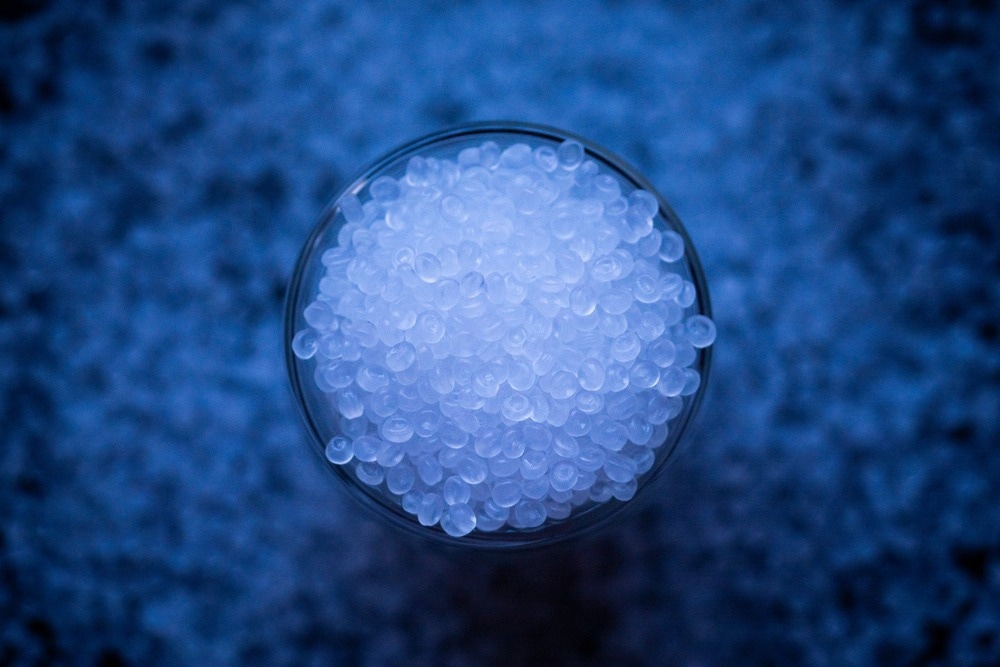
Image Credit: Koray Akar/Shutterstock.com
How Polymer Properties are Tested
With the knowledge that these polymers will be subjected to varying temperatures throughout their life cycle (be it through transportation, use, or storage), they are tested at very high and very low temperatures. The fragile sections of all polymeric material are found in the secondary bonds between polymer subunits. As the temperature increases, these subunits will vibrate at greater speeds because of their higher kinetic energy. This will weaken van-der-walls forces (and sometimes dipole-dipole forces) between these monomeric units. This is why these materials should first be subjected to these temperatures for testing.
The properties and stability of polymers are likewise tested through the uptake of nitrogen gas at a low temperature of approximately 196 degrees centigrade. Temperature decreases will lead to lesser mobility, which is why plastics become brittle at lower temperatures.
Sources:
- Gkika, D. A., Filiz, V., Rangou, S., Kyzas, G. Z., & Mitrοpoulos, A. C. (2022). Cost Profile of Membranes That Use Polymers of Intrinsic Microporosity (PIMs). Membranes, 12(4), 433. https://doi.org/10.3390/membranes12040433
- Max J. H. Worthington,a Renata L. Kuceraa and Justin M. Chalker, (2017) Green chemistry and polymers made from sulfur. Green Chem.,19, 2748-2761 DOI https://doi.org/10.1039/C7GC00014F
- Scholes, C. A., & Kanehashi, S. (2019). Polymer of Intrinsic Microporosity (PIM-1) Membranes Treated with Supercritical CO₂. Membranes, 9(3), 41. https://doi.org/10.3390/membranes9030041
- Adams, F., & Adriaens, M. (2020). The metamorphosis of analytical chemistry. Analytical and bioanalytical chemistry, 412(15), 3525–3537. https://doi.org/10.1007/s00216-019-02313-z
- Ghaffar A, Schoenmakers PJ, van der Wal S. Methods for the chemical analysis of degradable synthetic polymeric biomaterials. Crit Rev Anal Chem. 2014;44(1):23-40. doi: 10.1080/10408347.2013.831729. PMID: 25391212.
Further Reading