Genomics is a relatively emerging field that has undergone exceptional development over the last decades. In agriculture, genomics research has enabled the identification of genes and genomic regions associated with phenotypic traits of agronomic importance. In medicine, this discipline offers new opportunities for improved diagnostics and treatment of genetic diseases.
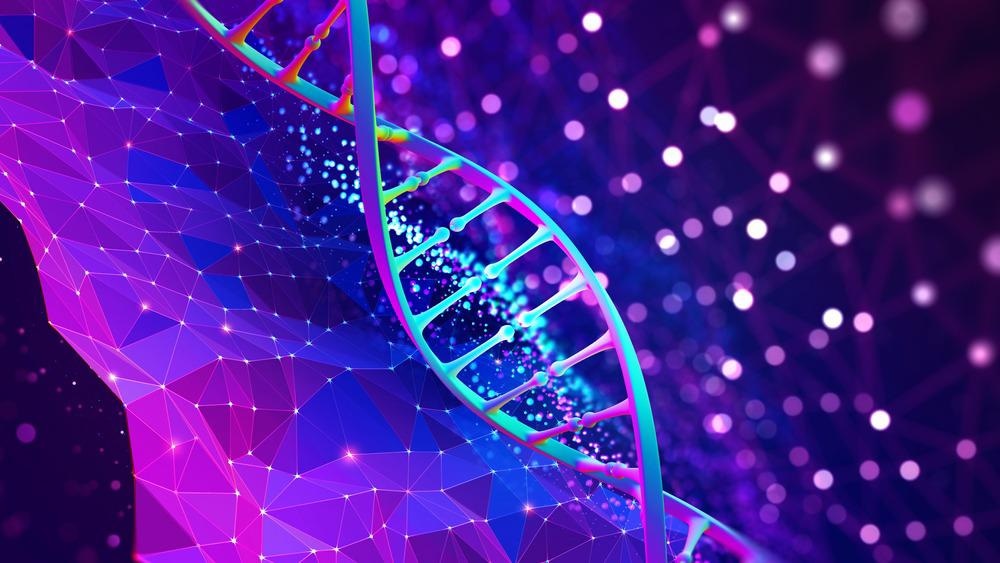
Image Credit: Yurchanka Siarhei/Shutterstock.com
Genomics is a scientific field that combines DNA-based technologies with bioinformatics/computational tools to sequence, assemble, and annotate genomic and transcriptomic (RNA) data. Genomics includes different molecular-based approaches such as cloning, sequencing, and recombinant DNA technology. This discipline studies the genome's structure and function to discover genes involved in any observable trait recognized as having underlying genetic causes.
The development of Genomics as an Experimental Science
Deoxyribonucleic acid (DNA) is a fundamental macromolecule for life whose primary role is to store genetic information that encodes for proteins, which can be stably transferred across generations from parents to offspring. A genome can then be defined as all of the genetic material (DNA) of a cell. The linear order of the nucleotides in DNA largely determines the type and amount of proteins encoded by a particular genome and roughly explains the whole physiological capability of an organism.
Genomics —the discipline that deals with genomes and DNA sequences— is now at the threshold of a revolution due to the development of next-generation sequencing platforms and other omics approaches. These platforms can handle long genomic sequences by breaking them into short pieces of DNA that can be sequenced by automatic sequence analyzers and assembled into whole chromosomes.
The access to whole-genome sequences has given birth to a new field of investigation called comparative genomics, which allows the identification of novel genes and validation of predicted ones. High throughput genomics technologies can be used to discover genes that play central roles in specific cellular processes and intergenic (non-coding) regions that regulate gene expression.
The recombinant DNA (rDNA) technique plays a crucial role in genomics. This methodology involves the insertion of a foreign piece of DNA into an autonomously replicating carrier, commonly known as a 'cloning vector.' A carrier molecule may consist of a fragment of viral DNA, bacterial plasmids, yeast artificial chromosomes, etc. The rDNA method makes it possible to construct gene and genomic libraries for further studies. A genomic library can then be cloned and maintained in a suitable cloning vector to be finally digested and sequenced by next-generation sequencing platforms that offer scalability and speed.
The emergence of more sophisticated and powerful computational tools has led to the development of an interdisciplinary field called bioinformatics. In genomics, bioinformatics tools can be used to assemble and analyze massive volumes of data. Over the last years, the development of new bioinformatic resources has dramatically increased the specificity for assembling and analyzing sequence data at an unprecedented depth and scale.
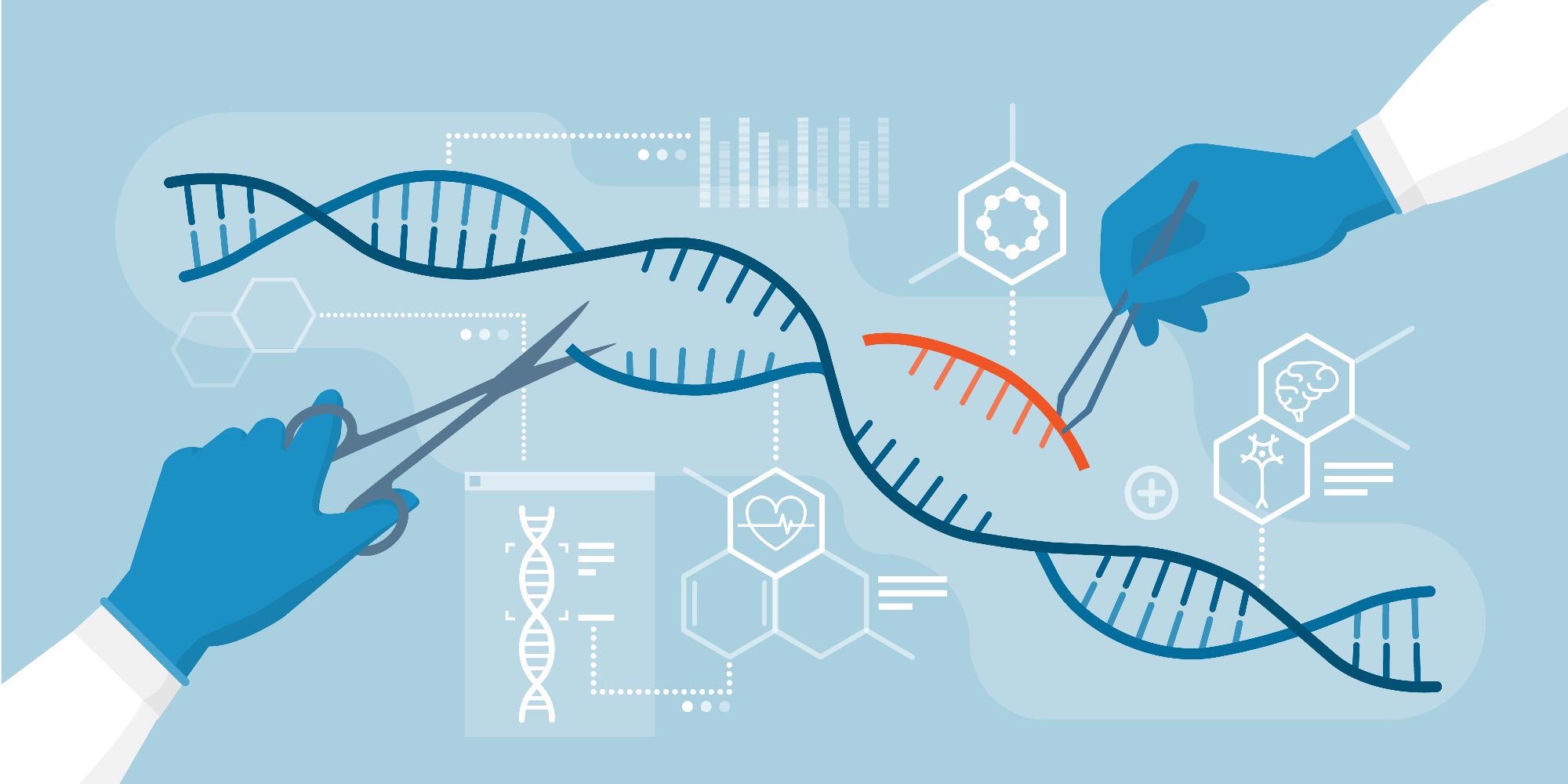
Image Credit: elenabsl/Shutterstock.com
What does the future of genomics look like?
The development of the latest technologies for the de novo sequencing, assembly and analysis of the sequence data allows for predicting a promising future for genomics. It is expected that such advances will also bring along new challenges. The first and most challenging issue is the design of bioinformatics methods for integrating omics data, which might help predict how a genome can shape multiple phenotypic traits in different environments.
As genetic engineering evolves, an increased number of advances helping to boost genomics research are emerging. The CRISPR-Ca9 system for efficient genomic engineering deserves a special mention. It is one of the most promising strategies for triggering targeted mutations at a genome-wide scale. CRISPR-Cas9 is a genome-editing tool that can be used to edit nucleotide sequences and thus explore how specific mutations at multiple loci can be associated with a particular phenotype in any cell line of choice.
How has Technology Transformed Genomics Research?
Sources:
- Collins, F. S., Doudna, J. A., Lander, E. S., & Rotimi, C. N. (2021). Human molecular genetics and genomics—important advances and exciting possibilities. New England Journal of Medicine, 384(1), 1-4.
- Edwards, D., Batley, J., & Snowdon, R. J. (2013). Accessing complex crop genomes with next-generation sequencing. Theoretical and Applied Genetics, 126(1), 1-11.
- Gatew, H., & Tarekegn, G. M. (2018). Next-generation sequencing platforms for latest livestock reference genome assemblies. African Journal of Biotechnology, 17(39), 1232-1240.
- Mantere, T., Kersten, S., & Hoischen, A. (2019). Long-read sequencing emerging in medical genetics. Frontiers in genetics, 10, 426.
- Metje-Sprink, J., Menz, J., Modrzejewski, D., & Sprink, T. (2019). DNA-free genome editing: past, present and future. Frontiers in Plant Science, 1957.
- Nemtsova, M. V., Kalinkin, A. I., Kuznetsova, E. B., Bure, I. V., Alekseeva, E. A., Bykov, I. I., ... & Strelnikov, V. V. (2020). Clinical relevance of somatic mutations in main driver genes detected in gastric cancer patients by next-generation DNA sequencing. Scientific reports, 10(1), 1-11.
- Ostrov, N., Beal, J., Ellis, T., Gordon, D. B., Karas, B. J., Lee, H. H., ... & Tekotte, H. (2019). Technological challenges and milestones for writing genomes. Science, 366(6463), 310-312.
Further Reading