Pyramid DNA is a group of small, tetrahedral DNA nanostructures. They possess different characteristics from linear DNA, facilitating their use in sensors, biological models, and drug delivery. This is achieved due to their thermostability, appreciable cell membrane penetration, and amenability to modification and conjugation. The advent of DNA origami has accelerated their generation. This process involves one long single-stranded DNA (ssDNA) of 7000 base pairs combined with hundreds of short ssDNA "staples" that can fold the long strand into complex structures.
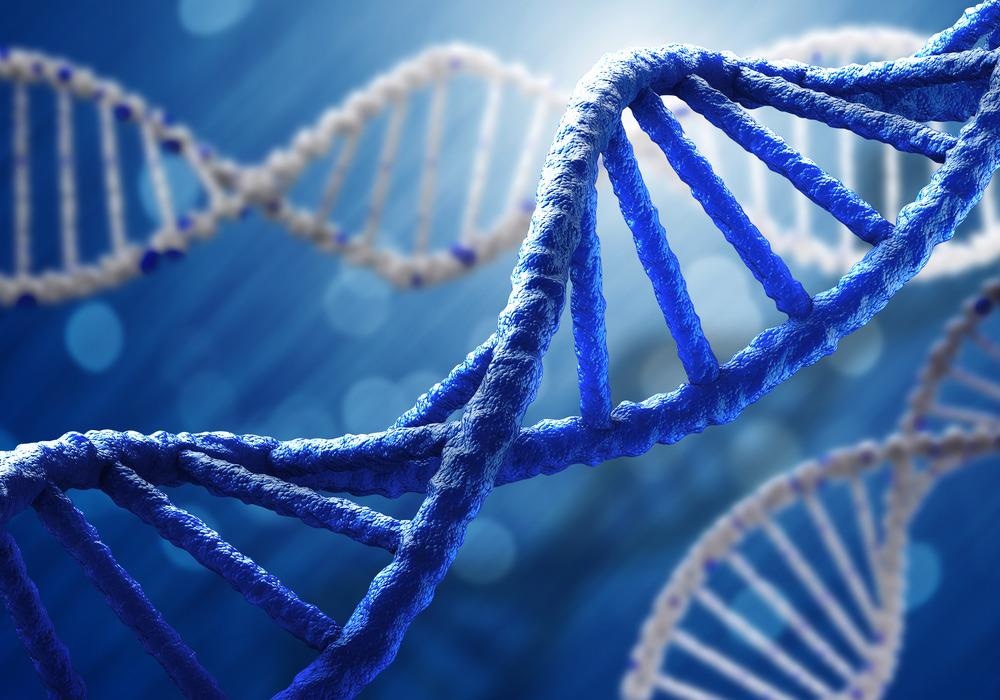
Image Credit: ESB Professional/Shutterstock.com
Generation
One of the most established and stable structures generated using DNA is the tetrahedral pyramid. Many methods have been adopted to generate these structures. Critical elements to keep in mind are the polarity of the DNA strands and the appropriate sequences to generate vertices. Older processes relied on synthetic DNA strands manipulated under certain conditions to form pyramids.
One method involved the heating of these strands in a salt solution to just below boiling point. This process is highly effective and rapid. It has been shown to be thermostable and capable of withstanding high pressure. Advancements in DNA nanotechnology allow for applied techniques that conjugate moieties to confer further characteristics.
One technique relies on the development of X-DNA structures. These hybridize and ligate due to complementary strands on the sticky ends of X-DNA. Gold particles can then be assembled onto the pyramids using electrostatic interactions, conferring therapeutic benefits.
The generation of short single-stranded oligonucleotides is often necessary to ligate the respective faces while conferring sufficient flexibility to act as a linker. The formation of pyramid DNA can quickly be confirmed using native Poly Acrylamide Gel Electrophoresis, which maintains the native 3D structure.
Drug delivery
One of the most exciting potential applications of pyramidal DNA is its therapeutic benefit as a means of delivering drugs and gene therapies. It possesses numerous benefits: high biocompatibility, biodegradability, low immunogenicity, and is easily manipulated to improve efficacy. Pyramid DNA nanostructures can be modified to allow the conjugation of nucleotide-based ligands such as siRNA, aptamers, etc. This was achieved using cancer cell receptor targeting aptamers.
Interestingly, DNA pyramids are capable of penetrating the cell membrane; this process is accelerated in the presence of aptamers. The process is specific to cancerous cell lines and is readily degraded in non-cancerous cell lines. One issue that needs to be addressed is stability in vivo; the cellular environment is rich in nucleases and other enzymatic components that could have harmful effects on the efficacy of the pyramid DNA.
Pyramid DNA also acts as a vehicle for drug delivery, including small molecules and peptide-based therapies. Doxorubicin, an anti-cancer drug, has been loaded onto these nanostructures, along with tumor-penetrating peptides, aptamers, or folic acid. This application demonstrated comparative efficacy at lower concentrations, improved uptake, and a more targeted approach. This process may also be capable of overcoming resistance associated with tumor-mediated efflux pump.
Drugs can be non-covalently associated or covalently drafted onto the DNA structure. The latter can facilitate a pro-drug delivery to the tumor or site of action to mitigate off-target effects.
DNA nanostructures can also present new avenues in the fight against antibiotic resistance. They have been used to transport GL13K, an antimicrobial agent, to assess the inhibition of different microbial species. It demonstrated an improved antimicrobial effect and protected it from degradation from proteases.
Antisense nucleic acid-based DNA nanostructures have been constructed that target methicillin-resistance Staphylococcus aureus cells. The antisense nucleic acid targeted a specific gene to silence it in a concentration-dependent manner. Treatments like these are more specific, present a lower risk of resistance, and maintain the microbiome's diversity.
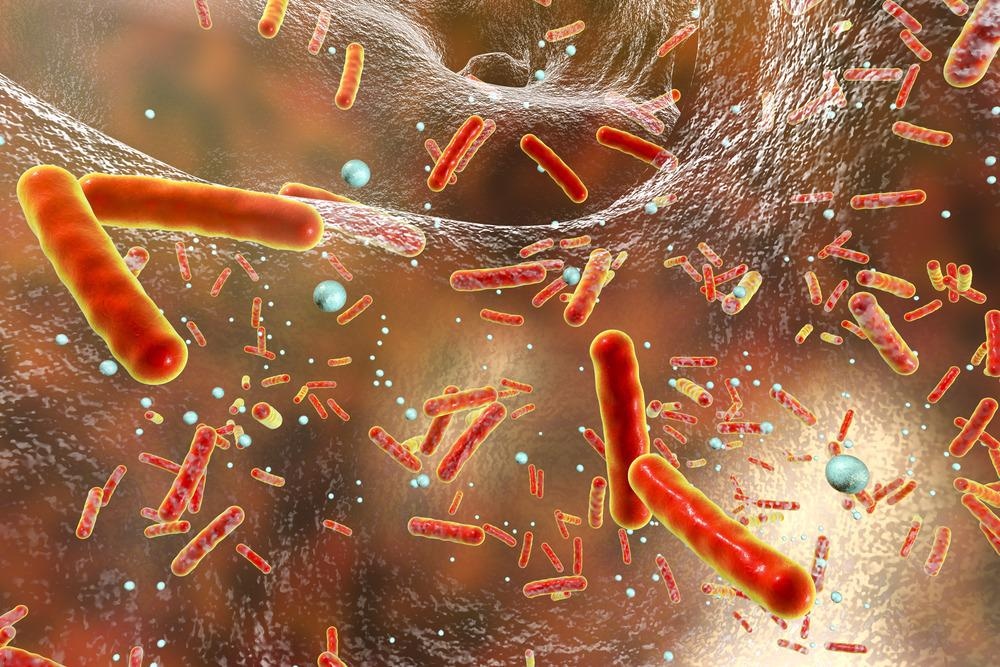
Image Credit: Kateryna Kon/Shutterstock.com
Sensors
Pyramidal DNA structures can not only deliver therapies; they pose the possibility of detecting perturbations in cellular processes. Modified amphiphilic pyramid DNA has demonstrated excellent efficacy as cell membrane anchors, facilitating cell-cell interactions in a tunable, regulated manner. This is critical for paracrine signaling, immunology, and membrane biology.
DNA nanostructures have also been used to emulate ligands to understand the complex molecular relationships between receptor and ligand. It has been utilized to investigate how the spatial distribution of ephrin-A5 affected the activation levels of the EphA2 receptor. It has also been applied to investigate how antigen distribution modulates B-cell activation.
These processes afford great sensitivity. This has been demonstrated in detecting Staphylococcus enterotoxin B (SEB). The nanostructure comprises three thiolated probes, an auxiliary probe, and an aptamer against SEB, assembled on a gold electrode. In the absence of SEB, the aptamer sits at the top of the assembled structure.
Electron transfer to a chemical, hexacyanoferrate, acts as a probe. This is impeded in the absence of SEB. In the presence of SEB, however, the aptamer binds to the enterotoxin, and the structure collapses to a 2D structure. This enables the transfer of an electron to hexacyanoferrate. This process is comparable to ELISA detection.
A similar process has been adopted for the detection of Ochratoxin A in food and serum samples. A DNA nanostructure was assembled with a fluorescent aptasensor. In the absence of the toxin, the structure remains intact, and fluorescent emission is strong. In the presence of the toxin, the aptasensor binds to it, resulting in a loss of emission.
Conclusion
Pyramid DNA nanostructures are stable, adaptable vehicles for therapeutics, modeling, and detection. They possess significant advantages: they are stable, accessible to human tissues, easily conjugated, less immunogenic, and scalable. Further investigations are necessary to assess the long-term implications of these structures in terms of bioavailability, surveillance, and degradation in vivo.
Sources:
- Chen, L. et al. (2021) 'Pharmaceutical applications of framework nucleic acids', Acta Pharmaceutica Sinica B. doi: https://doi.org/10.1016/j.apsb.2021.05.022.
- Chen, X. et al. (2018) 'Impedimetric determination of Staphylococcal enterotoxin B using electrochemical switching with DNA triangular pyramid frustum nanostructure', Microchimica Acta, 185(10), p. 460. doi: 10.1007/s00604-018-2983-3.
- Keum, J.-W., Ahn, J.-H. and Bermudez, H. (2011) 'Design, Assembly, and Activity of Antisense DNA Nanostructures', Small, 7(24), pp. 3529–3535. doi: https://doi.org/10.1002/smll.201101804.
- Li, J. et al. (2019) 'Cell-Membrane-Anchored DNA Nanoplatform for Programming Cellular Interactions', Journal of the American Chemical Society, 141(45), pp. 18013–18020. doi: 10.1021/jacs.9b04725.
- Nameghi, M. A. et al. (2016) 'A fluorescent aptasensor based on a DNA pyramid nanostructure for ultrasensitive detection of ochratoxin A', Analytical and Bioanalytical Chemistry, 408(21), pp. 5811–5818. doi: 10.1007/s00216-016-9693-7.
- P., G. R. et al. (2005) 'Rapid Chiral Assembly of Rigid DNA Building Blocks for Molecular Nanofabrication', Science, 310(5754), pp. 1661–1665. doi: 10.1126/science.1120367.
- Setyawati, M. I. et al. (2014)' Novel Theranostic DNA Nanoscaffolds for the Simultaneous Detection and Killing of Escherichia coli and Staphylococcus aureus', ACS Applied Materials & Interfaces, 6(24), pp. 21822–21831. doi: 10.1021/am502591c.
- Song, J. et al. (2017) 'Electrostatic interaction driven gold nanoparticle assembly on three-dimensional triangular pyramid DNA nanostructures', New Journal of Chemistry, 41(18), pp. 9590–9593. doi: 10.1039/C7NJ01944K.
- Veneziano, R. et al. (2020) 'Role of nanoscale antigen organization on B-cell activation probed using DNA origami', Nature Nanotechnology, 15(8), pp. 716–723. doi: 10.1038/s41565-020-0719-0.
- Zhang, Y. et al. (2018) 'Inhibiting Methicillin-Resistant Staphylococcus aureus by Tetrahedral DNA Nanostructure-Enabled Antisense Peptide Nucleic Acid Delivery', Nano Letters, 18(9), pp. 5652–5659. doi: 10.1021/acs.nanolett.8b02166.
Further Reading