Quantum mechanics can be used to understand those biological processes that cannot be accurately described by the classical laws of physics. Quantum biology applies quantum theory to explain aspects of biology where classical physics fails to provide an answer.
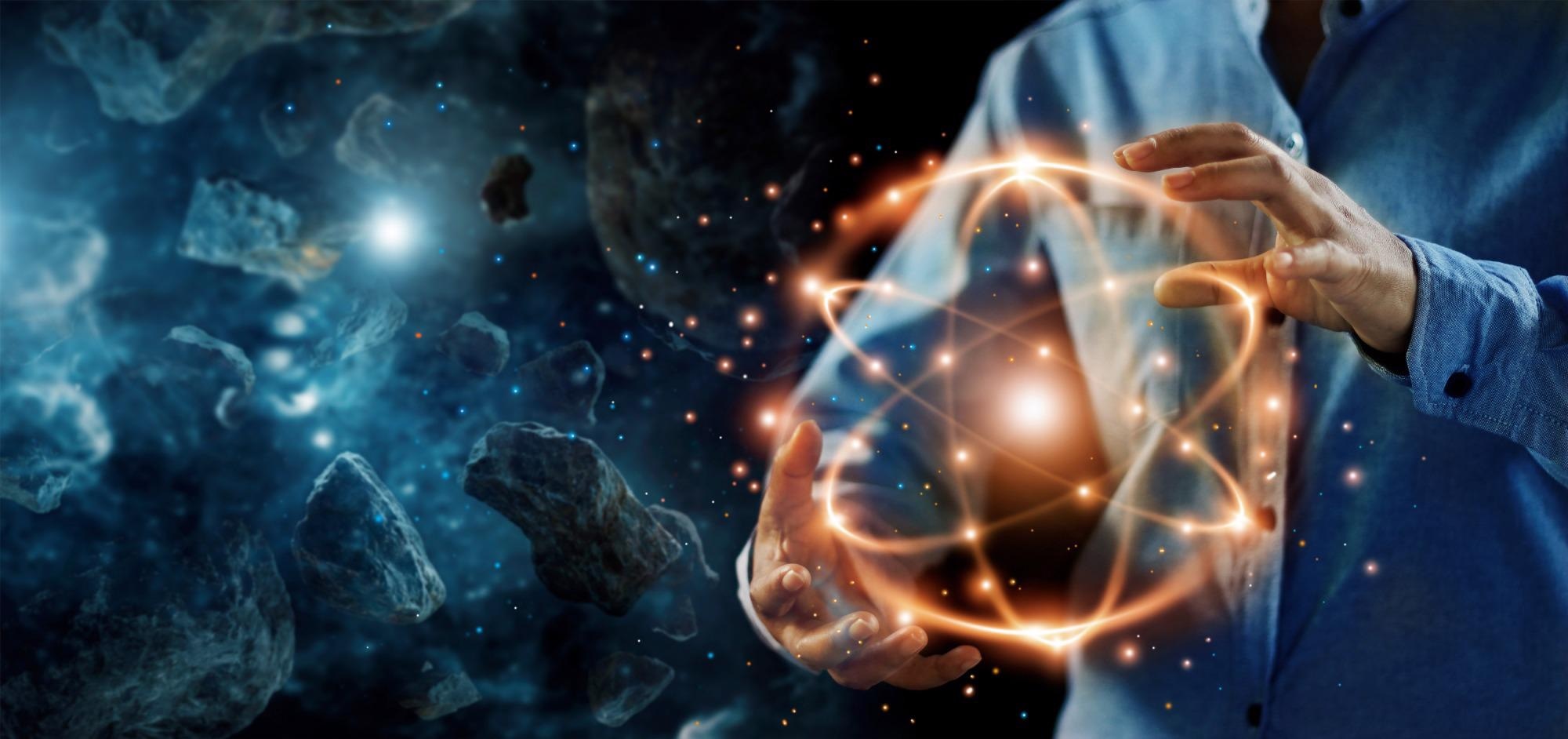
Image Credit: PopTika/Shutterstock.com
This emerging area of science is considered to be relatively new, although first considerations on how nature could recur to quantum mechanics to gain a biological advantage were already proposed in the first half of the twentieth century, right when quantum mechanics was in its blooming phase.
Only over the last decade though, have the advances in experimental techniques such as ultrafast spectroscopy, single-molecule spectroscopy, and single-particle imaging helped many researchers in developing the field of quantum biology.
It has become possible to study biological dynamics on increasingly smaller time scales, showing how certain processes, necessary for the function of living systems, require quantum mechanics to explain them. This includes phenomena like quantum coherence and tunneling.
Quantum biology has in fact revealed that processes such as photosynthesis, vision, and enzyme catalysis manifest quantum effects. It is fascinating how phenomena that were previously thought to be exclusive of atomic and subatomic systems, and that could only work at extremely low temperatures, can now be observed in some of the mechanisms responsible for life.
The strong interest in this new field is further enhanced by the potentially huge technological impacts. Quantum biology could in fact boost the development of biologically inspired nanotechnologies, particularly concerning sensing, health, and information technologies.
Quantum Coherent Energy Transfer in Photosynthesis
One of the most well-studied examples of quantum effects observed in biological processes is associated with photosynthesis. There is experimental evidence that quantum coherence takes place inside the bacteria performing photosynthesis.
Chlorophyll molecules are equipped with light-harvesting antennas that capture photons, which are then delivered to the reaction center where they are turned into energy. This process has an efficiency close to 100%, where almost every photon that is captured is transferred successfully to the reaction center.
Initially, it was thought that the photon transfer followed random paths. Quantum biology has then evidenced that in order to optimize the most efficient way to reach the reaction center, photons follow multiple pathways simultaneously via quantum coherence.
There is a lot of interest in explaining and reproducing such a process. A detailed understanding of photosynthesis on a microscopic scale could in fact lead to the design of biologically inspired artificial photosynthetic systems that can exploit sunlight energy more efficiently.
Energy and Charge Transfer via Quantum Tunnelling
Tunneling is an archetypal quantum effect, very well-studied in quantum physics. Quantum biology has shown that some proteins perform redox reactions using long-range electron tunneling, with reaction centers distant up to 30 Å.
The process shows exponential distance and temperature dependence, in agreement with a single-step tunneling mechanism. This type of phenomenon on a biological system is remarkable considering that electron tunneling over such a long distance would be impossible in a vacuum.
There is also evidence that some enzymes recur to quantum tunneling to perform proton transfer to carry out their catalytic reactions. It was observed that protons can be transferred from one part of the molecule to another.
There are also indications suggesting that quantum tunneling is thought to play a role in DNA mutations. The two strands of DNA are held together by hydrogen bonds established between complementary nucleobases. During the tautomerization of DNA bases from their common imino forms to the rare enol forms, two opposing nucleobases can exchange protons.
During the replication phase, the DNA strand can separate while the hydrogen atoms are in the wrong positions. This can lead to incorrect base pairs and eventually to a mutation. Therefore, random point mutations might have a quantum origin.
How Quantum Biology Might Explain Life’s Biggest Questions | Jim Al-Khalili | TED Talks
Influencing the Birds’ Biological Compass During Migration
It is now well established that some bird species use the Earth’s magnetic field to navigate during migration, thanks to a phenomenon known as magnetoreception. The mechanism behind avian magnetoreception is a great unanswered question, that could find an answer thanks to quantum biology.
The navigation sense of some birds, like the European Robin, is dependent on the presence of certain frequencies of ambient light and is sensitive to changes in the intensity of the external magnetic. Explanation on how this biological compass is regulated has been proposed with the radical-pair mechanism.
Cryptochromes, light-sensitive proteins inside the robin’s retina, contain a radical pair of electrons that are entangled – meaning that they are somehow in contact with each other although far apart. Response to light causes photoexcitation of the radical pair, which consequently oscillates between singlet and triplet spin states. The mixing of singlet-triplet states is what is influenced by the very weak Earth’s magnetic field.
Theoretical and experimental investigations suggest that the disruption due to external fields is a quantum effect, and it represents another great example of how quantum mechanics could be involved in biological processes. However, this is still speculative at the moment due to challenges associated with reproducing the conditions where cryptochromes, or similar molecules, show sensitivity to very weak magnetic fields.
Sources:
- Mcfadden, J. & Al-Khalili, J. (2018). The origins of quantum biology. Proc Math Phys Eng Sci, 474, 20180674.10.1098/rspa.2018.0674
- Marais, A., Adams, B., Ringsmuth, A. K., Ferretti, M., Gruber, J. M., Hendrikx, R., Schuld, M., Smith, S. L., Sinayskiy, I., Kruger, T. P. J., Petruccione, F. & Van Grondelle, R. (2018). The future of quantum biology. J R Soc Interface, 15.10.1098/rsif.2018.0640
- Lambert, N., Chen, Y.-N., Cheng, Y.-C., Li, C.-M., Chen, G.-Y. & Nori, F. (2012). Quantum biology. Nature Physics, 9, 10-18.10.1038/nphys2474
Further Reading