Hamamatsu Photonics has used its proprietary camera design technology to create high-sensitivity, low-noise cameras since the 1980s. The company has also consistently contributed to advancing cutting-edge scientific and technological research. Using the proprietary design technology and state-of-the-art manufacturing processes, Hamamatsu developed the ORCA-Quest, a camera with a qCMOS image sensor. Furthermore, by resolving photon numbers, it is the first camera in the world to accomplish the pinnacle of quantitative imaging.
The ORCA-Quest 2 qCMOS camera is the upgraded version of the ORCA-Quest, featuring enhanced sensitivity in the ultraviolet spectrum and faster readout speeds in the ultra-low-noise scan mode.
Evolution From ORCA-Quest
Faster Ultra Quiet Scan Mode
ORCA-Quest has reached the stage where photon number resolving is possible because of its ultra-low noise characteristic in ultra-quiet scan mode. However, this availability was restricted for users because the ultra-low noise was only available when the camera was operating at five frames per second (in full resolution).
By maximizing sensor operation, ORCA-Quest 2 has attained a five-fold increase in framerate while maintaining a comparable ultra-low noise characteristic. The majority of users can now access the photon number resolving feature.
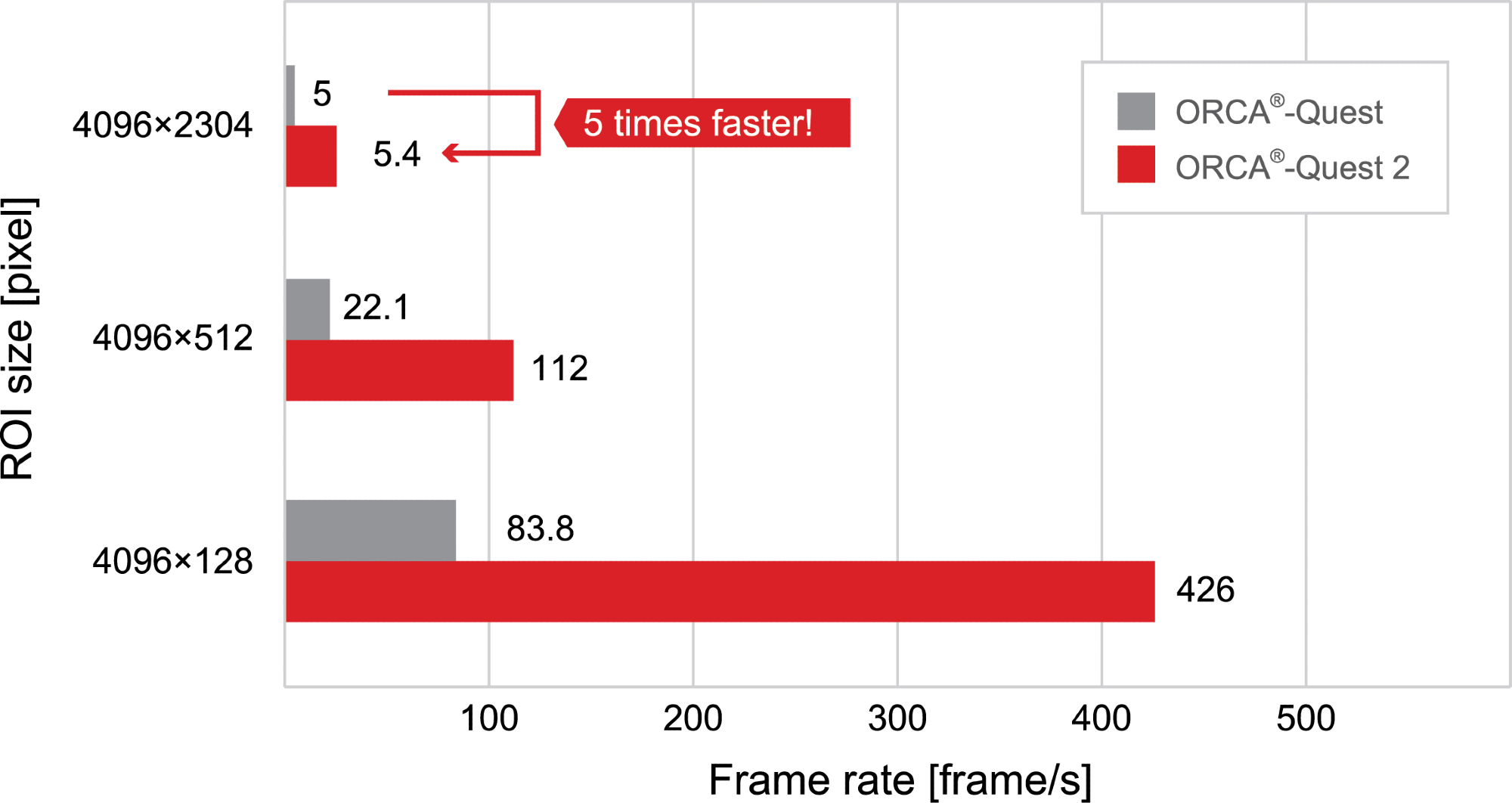
Image Credit: Hamamatsu Photonics Europe GmbH
UV QE Improvement
When considering the UV region between 280 and 400 nm, ORCA-Quest demonstrated a higher quantum efficiency (QE) than most traditional scientific cameras.
Motivated by market demands, ORCA-Quest 2 has optimized the AR coating of the sensor window to achieve even higher UV QE while maintaining the visible and near-infrared wavelength region unchanged. The QE enhancement increases the ORCA-Quest series' adaptability to various applications, including trapped ion quantum experiments.
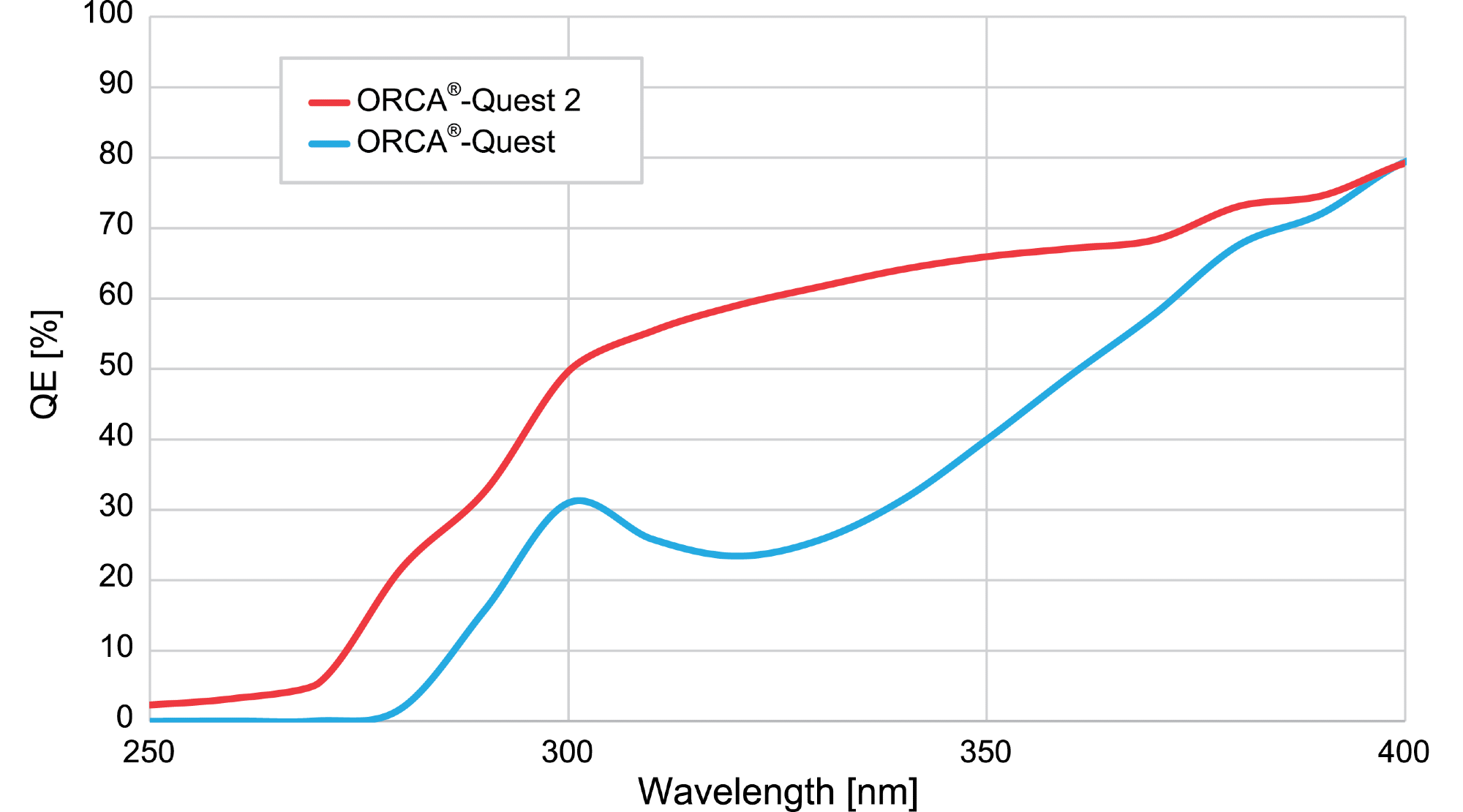
Image Credit: Hamamatsu Photonics Europe GmbH
Four Key Features
Extreme Low-Noise Performance
ORCA-Quest 2 has been designed and optimized for every sensor aspect, from structure to electronics, to detect weak light with high signal-to-noise. The latest CMOS technology has been employed in the development of the camera and in the creation of custom sensors, resulting in an exceptionally low noise performance of 0.30 electrons.
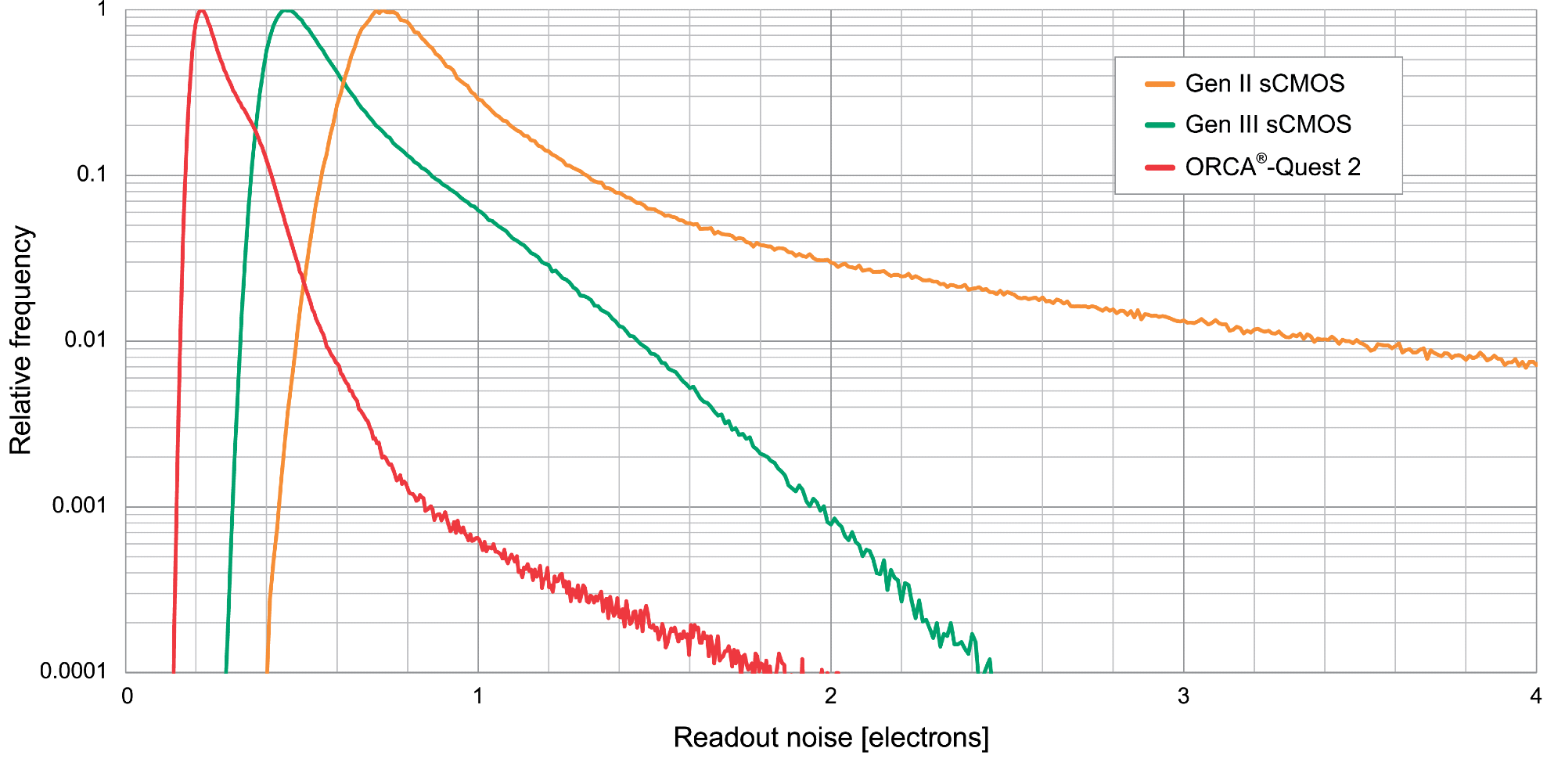
Image Credit: Hamamatsu Photonics Europe GmbH
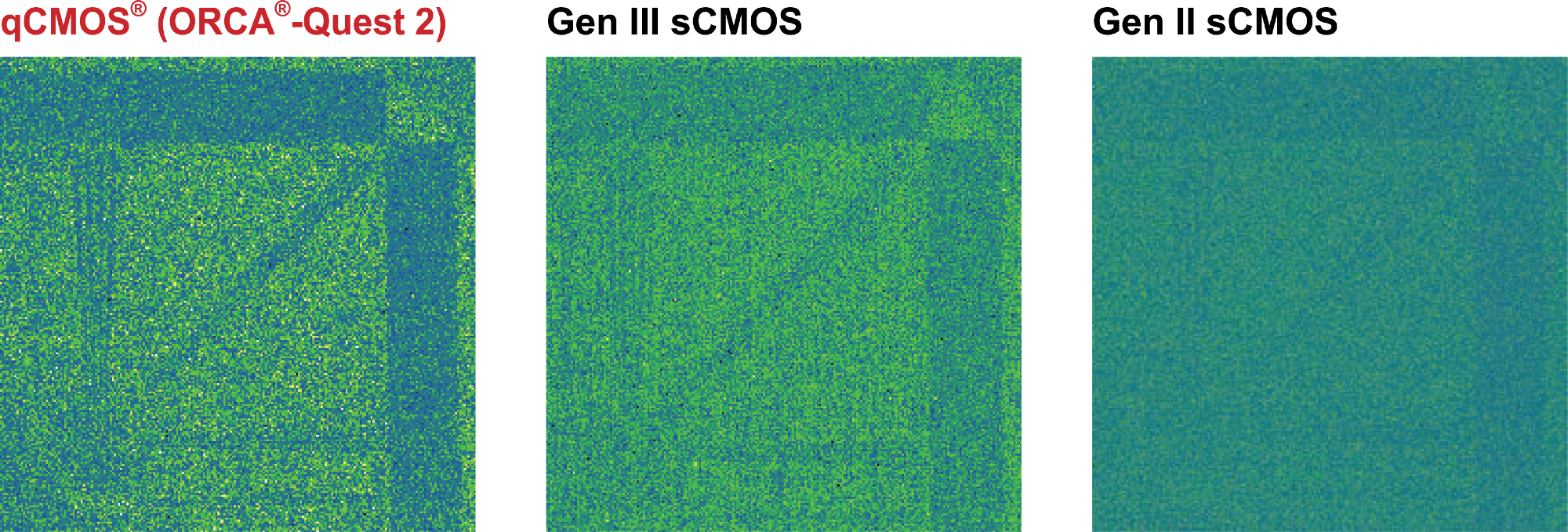
Comparison of average 1 photon per pixel image (pseudo-color). Exposure time: 200 ms, LUT: minimum to maximum value, and Comparison area: 512 pixels × 512 pixels. Image Credit: Hamamatsu Photonics Europe GmbH
Realization of Photon Number Resolving (PNR) Output
Light is made up of numerous photons. On the sensor, photons are transformed into electrons, called photoelectrons. A technique called "photon number resolving" uses photoelectrons to measure light precisely. Camera noise must be smaller than the photoelectron signal to count these photoelectrons.
Counting photoelectrons using conventional sCMOS cameras is challenging because they produce a small readout noise that is nevertheless larger than the photoelectron signal. The ORCA-Quest 2 counts photoelectrons using cutting-edge camera technology and provides an extremely low readout noise of 0.27 electrons rms (@Ultra quiet scan), stability over temperature and time, personalized calibration, and real-time pixel value correction.
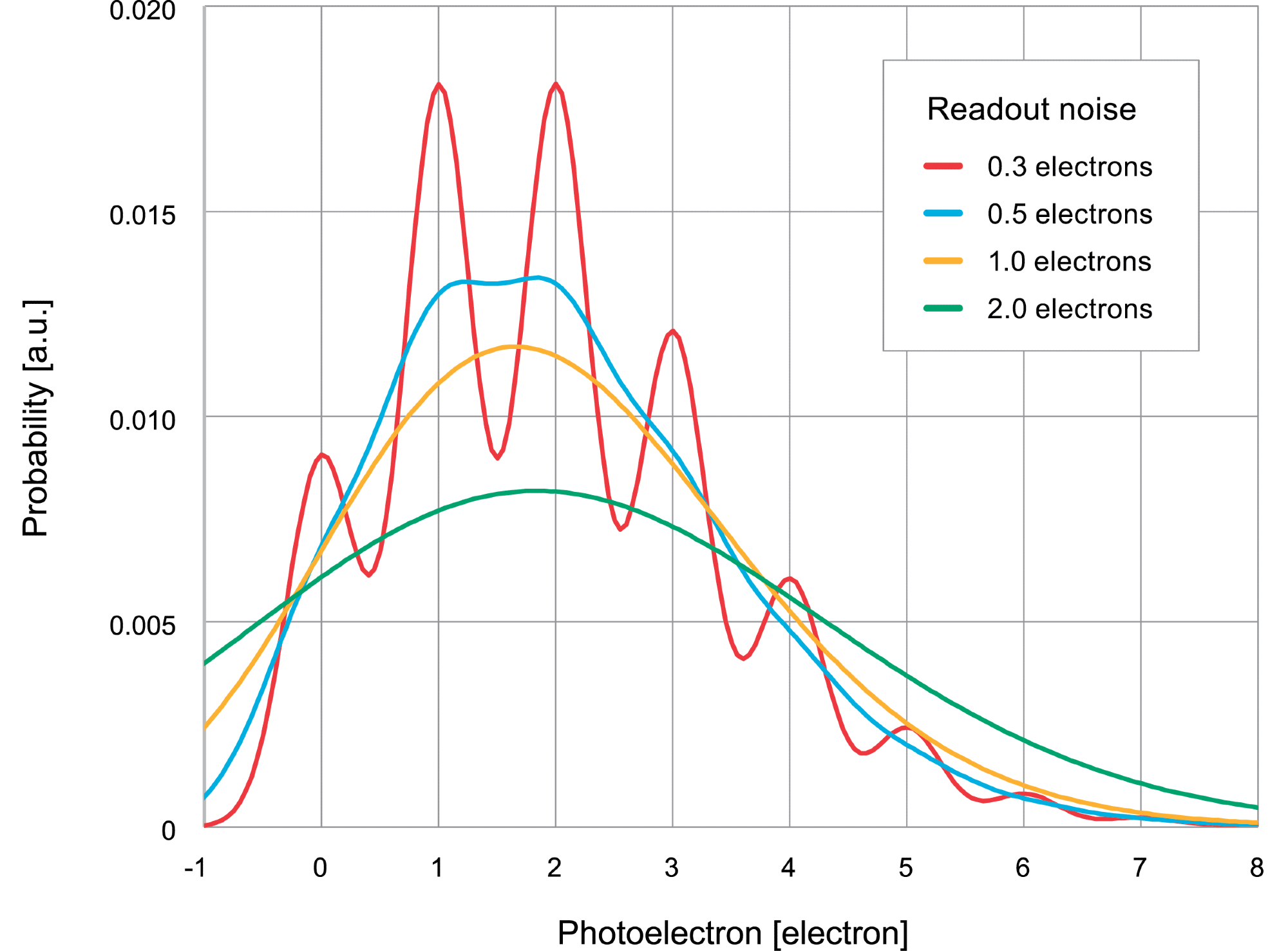
Simulation data of photoelectron probability distribution(Average number of photoelectrons generated per pixel: 2 electrons). Image Credit: Hamamatsu Photonics Europe GmbH
The ultimate in quantitative imaging by ORCA-Quest qCMOS camera
The ultimate in quantitative imaging is the ORCA-Quest qcMOS camera. Video Credit: Hamamatsu Photonics Europe GmbH
Back-Illuminated Structure and High-Resolution
High QE is attained by using a back-illuminated structure, which is necessary for high photon detection efficiency. Because there is no pixel separation in conventional back-illuminated sensors, crosstalks arise between pixels, and resolutions are typically lower than those of front-illuminated sensors. The ORCA-Quest 2 qCMOS sensor features a trench structure in each pixel to minimize crosstalk and a back-illuminated structure to achieve high quantum efficiency.
What is a Trench Structure?
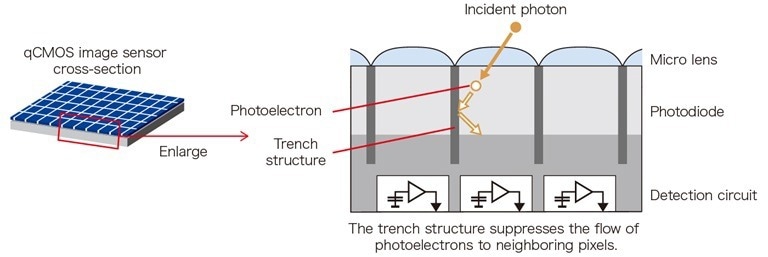
Image Credit: Hamamatsu Photonics Europe GmbH
Measurement Result of MTF
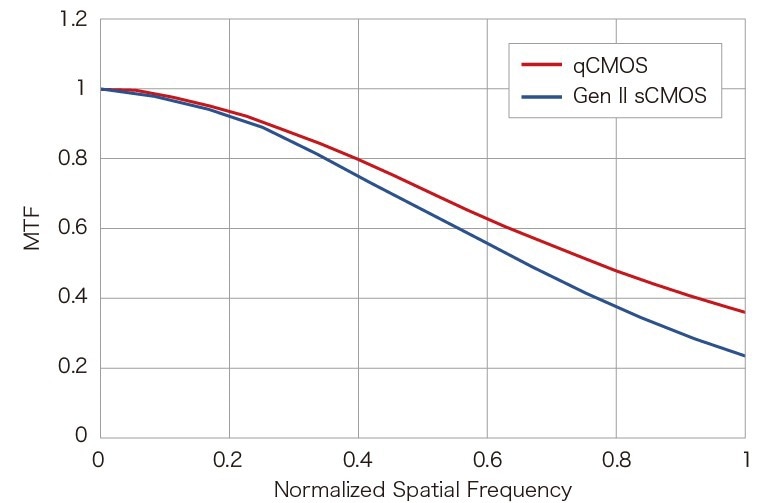
Modulation Transfer Function (MTF) is a type of resolution evaluation. It is the value of how accurately the contrast of an object can be reproduced. Image Credit: Hamamatsu Photonics Europe GmbH
Realization of a Large Number of Pixels and High-Speed Readout
ORCA-Quest 2's 9.4 megapixels (4096 (H) × 2304 (V)) allow for extremely low noise. More objects can be recorded with ORCA-Quest than traditional scientific cameras, such as the GenⅡ sCMOS and the EM-CCD camera.
ORCA-Quest 2 performs exceptionally well in terms of readout speed. To compare scientific cameras, Hamamatsu uses “data rate (number of pixels × frame rate),” which is the number of pixels a camera reads out in a second. Compared to traditional sCMOS cameras, ORCA-Quest 2 with Standard scan achieves higher data rates even with lower readout noise. Compared to single photon counting imaging by EM-CCD cameras, ORCA-Quest 2 with Ultraquiet scan realizes photon number resolving imaging at a data rate that is ten times faster.
Comparison of Pixel
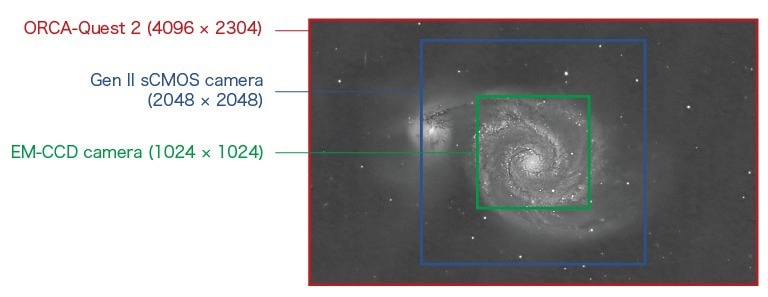
Image Credit: Hamamatsu Photonics Europe GmbH
Comparison of Data Rate
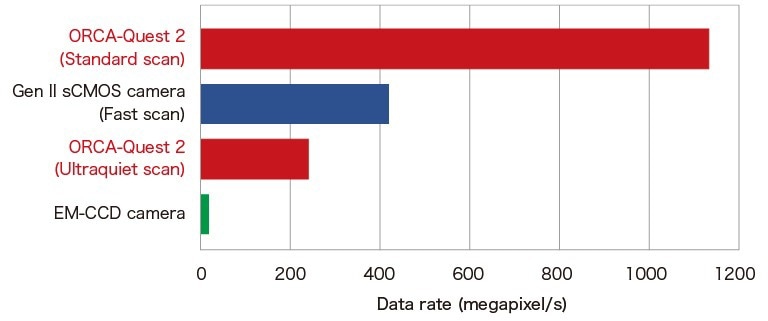
Image Credit: Hamamatsu Photonics Europe GmbH
Camera Articles
qCMOS Camera vs. EM-CCD Camera – Performance Comparison of Photon Counting Cameras
The qCMOS camera is an ultra-sensitive camera with exceptionally low noise performance, providing the best possible quantitative imaging. It is necessary to determine which camera is most appropriate for the application when comparing a qCMOS and an EM-CCD camera.
Applications
Quantum Technology
Neutral Atom, Trapped Ion
To be used as Qubits for quantum computing, neutral atoms and ions are arranged in an array one after the other. Users can determine the qubit states by observing the fluorescence from each of them. Photodetectors with extremely low noise and high speed are required after the fluorescence measurement, which must be completed quickly. With extremely low noise characteristics and fast readout, ORCA-Quest 2 can perform both state detection for each qubit and diagnosis of the entire qubit array. A large range of wavelengths for major atom and ion species are covered by the QE.
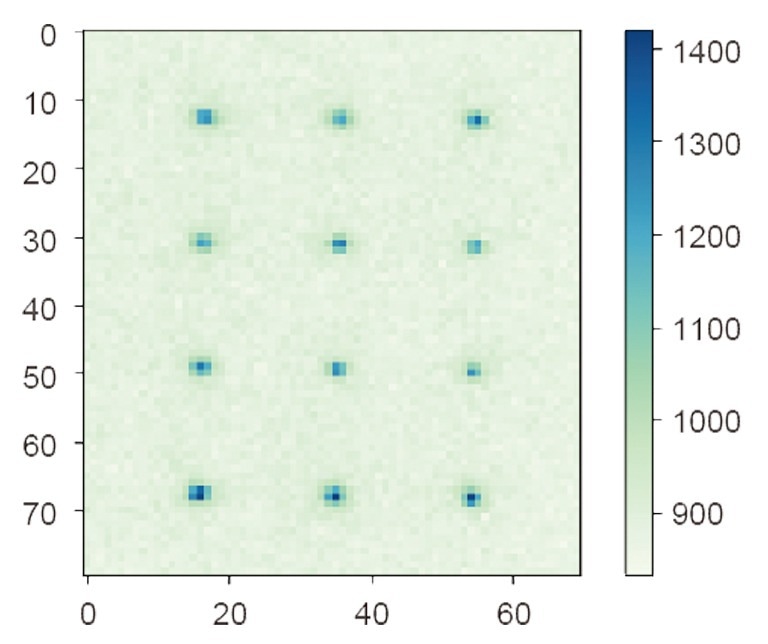
Fluorescence imaging of Rb atom array with ORCA-Quest. Image Credit: Takashi Yamamoto and Asst. Prof. Toshiki Kobayashi, Osaka University
Quantum Optics
In quantum optics, single photon sources harness the quantum nature of individual photons. Quantum optics research also employs single photon counting detectors, and there is a growing demand for photon number-resolving detectors to differentiate between varying numbers of photons detected. The emergence of photon-counting cameras, a novel concept in camera technologies, is anticipated to facilitate discoveries in this field.
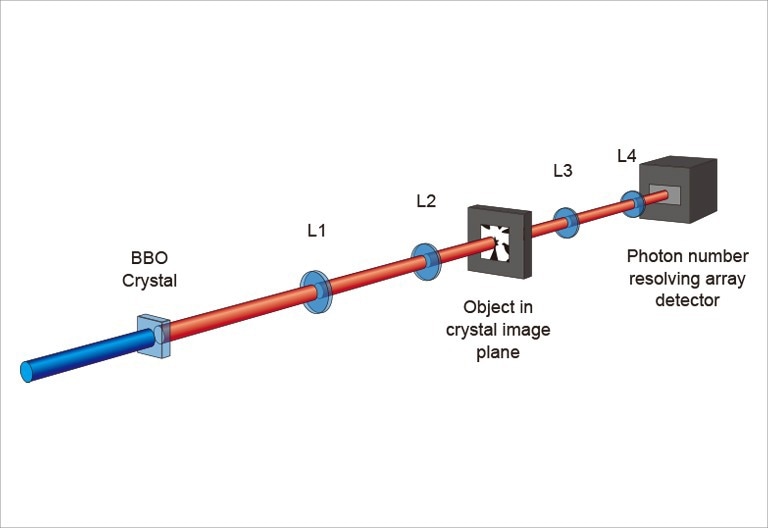
Experimental setup of Quantum imaging with ORCA-Quest. Image Credit: Miles Padgett, University of Glasgow
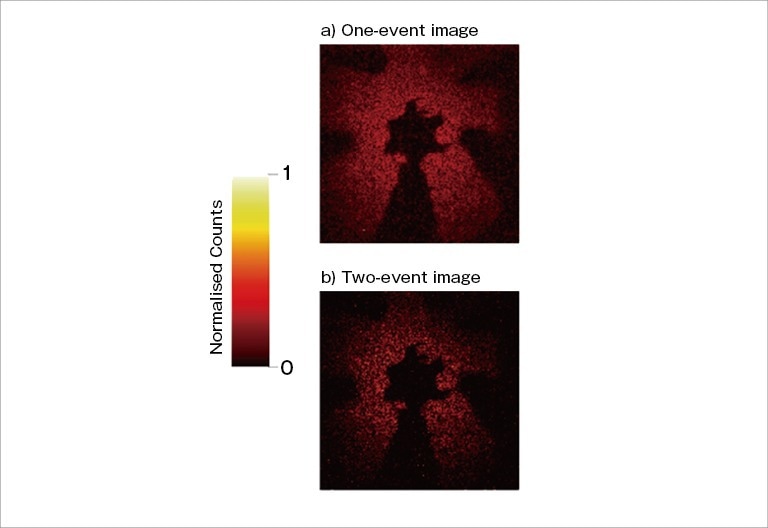
Images of Quantum imaging with ORCA-Quest. Image Credit: Miles Padgett, University of Glasgow
Life Science
Super-Resolution Microscopy
“Super-resolution microscopy” describes a group of techniques to obtain a microscope image with a spatial resolution greater than the diffraction limit. Scientific cameras that combine tiny pixel sizes and extremely low noise are required to achieve higher resolution for super-resolution microscopy.
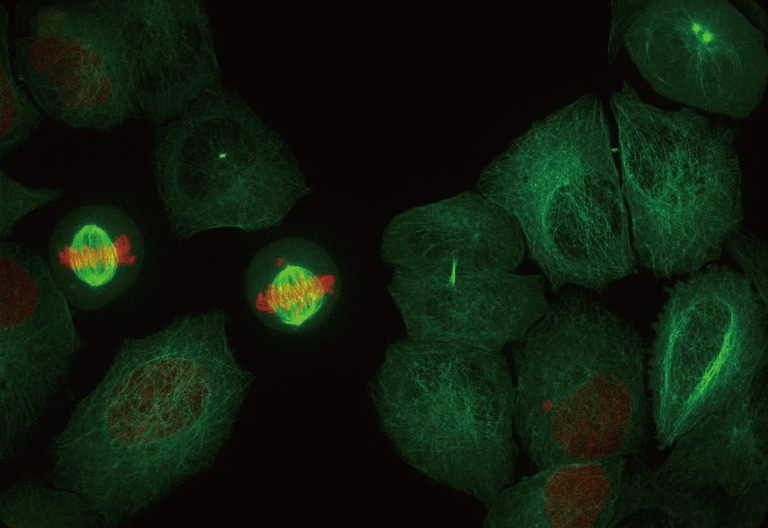
Super-resolution images from ORCA-Quest. qCMOS camera/4.6 μm pixel size. Image Credit: Steven Coleman at Visitech International with their VT-iSIM, high-speed super-resolution live cell imaging system.
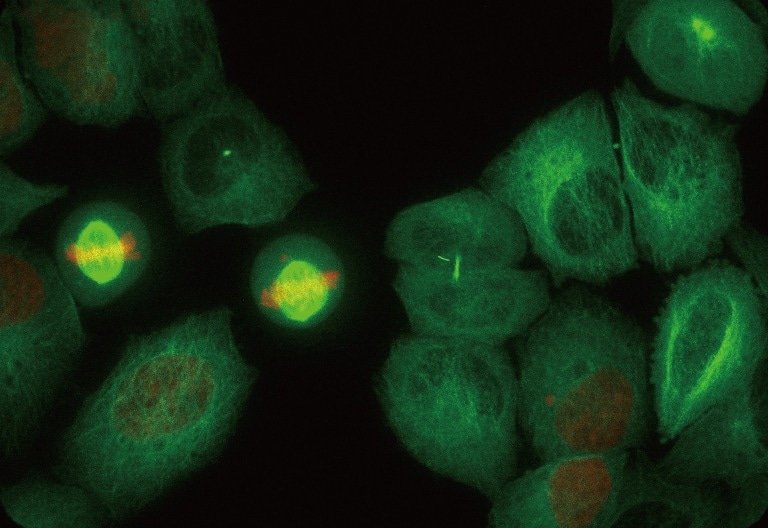
Super-resolution images from ORCA-Fusion. Gen III sCMOS camera/6.5 μm pixel size. Image Credit: Steven Coleman at Visitech International with their VT-iSIM, high-speed super-resolution live cell imaging system.
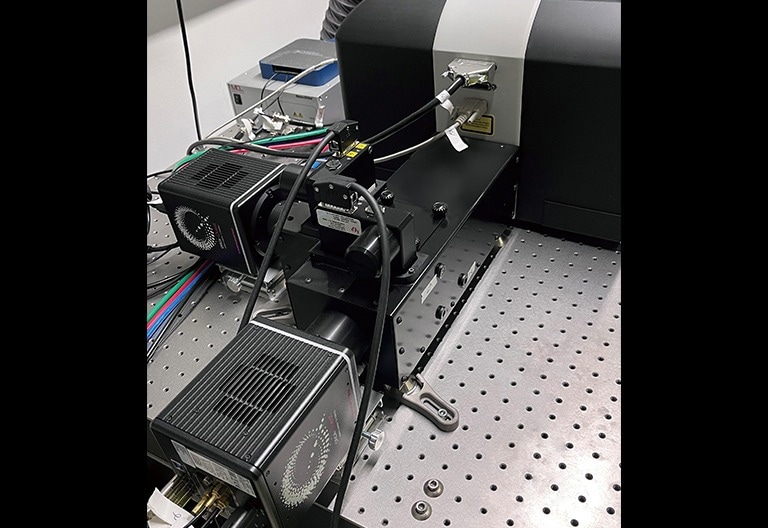
Experimental setup with ORCA-Quest. Image Credit: Steven Coleman at Visitech International with their VT-iSIM, high-speed super-resolution live cell imaging system.
Bioluminescence
Bioluminescence microscopy has garnered attention due to its distinct advantages over conventional fluorescence microscopy, notably the absence of the need for excitation light. However, its primary drawback lies in its extremely low light intensity, leading to prolonged exposure and diminished image quality. Bioluminescence research necessitates highly sensitive cameras, particularly for long exposures, to effectively capture and analyze data.
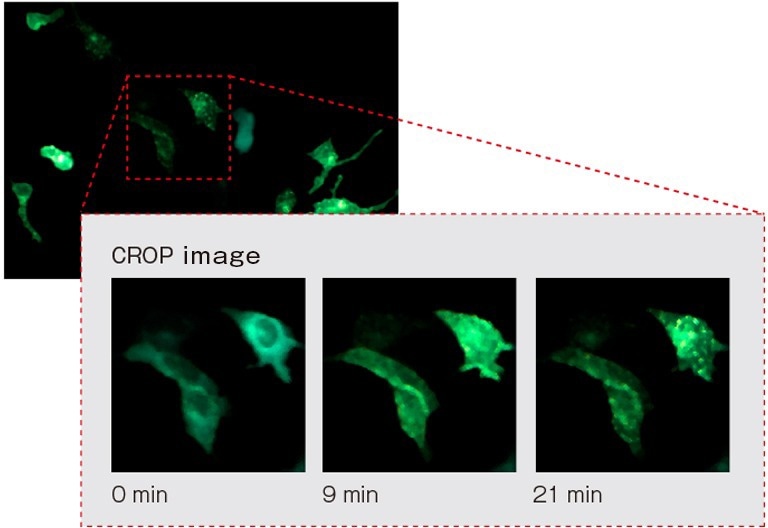
NanoLuc fusion protein ARRB2 and Venus fusion protein V2R are nearby, and BRET is occurring. Overall image in the field of view(Objective: 20×; Exposure Time: 30sec; Binning: 4 × 4). Image Credit: Dr.Masataka Yanagawa, Department of Molecular & Cellular Biochemistry
Appearance of the Microscope System
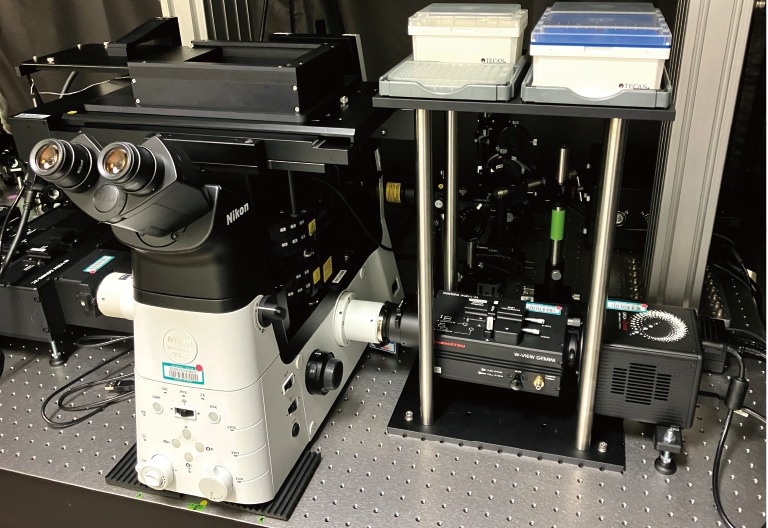
Image Credit: Dr.Masataka Yanagawa, Department of Molecular & Cellular Biochemistry Graduate School of Pharmaceutical Science, Tohoku University
Delayed Fluorescence in Plants
Plants gradually emit a minute fraction of the light energy they absorb during photosynthesis, a phenomenon termed delayed fluorescence. Detecting this subtle light allows for observing the impacts of chemicals, pathogens, environmental factors, and other plant stressors.
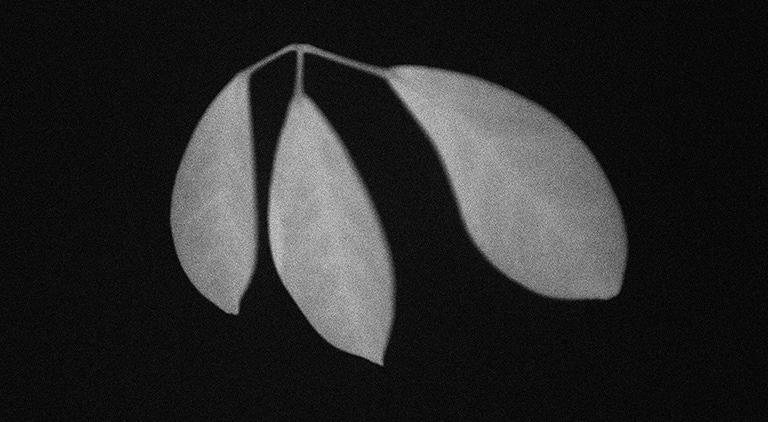
Delayed fluorescence of ornamental plants (exposure for 10 seconds after 10 seconds of excitation light quenching). Image Credit: Hamamatsu Photonics Europe GmbH
Astronomy
Lucky Imaging
Turbulence in the atmosphere can cause a star's image to become blurry when viewed from the ground, making it much harder to take sharp pictures of stars. With short exposures and favorable atmospheric conditions, clear images can occasionally be captured. Consequently, lucky imaging involves acquiring numerous images and selectively integrating only the clearest ones while aligning them, mitigating the effects of atmospheric turbulence.
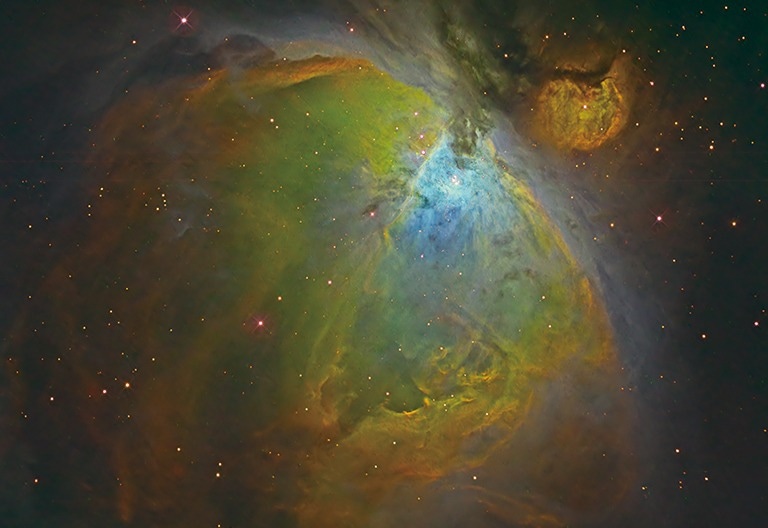
Orion Nebula (Color image with 3 wavelength filters). Image Credit: Hamamatsu Photonics Europe GmbH
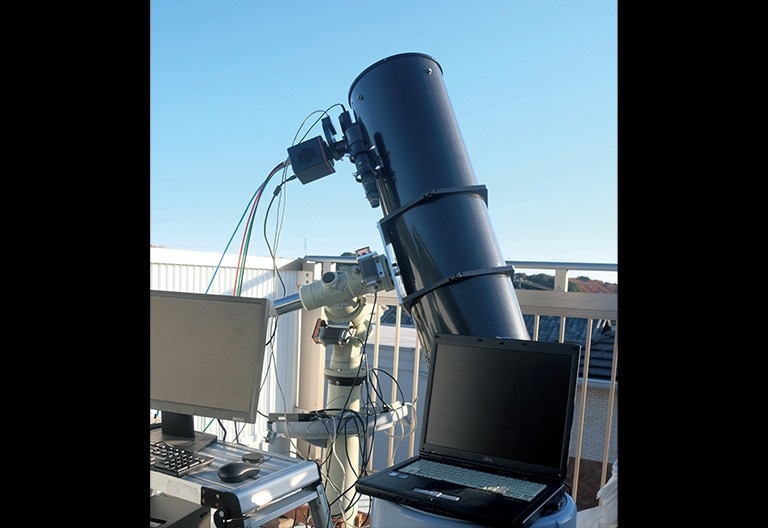
Imaging setup. Image Credit: Hamamatsu Photonics Europe GmbH
Adaptive Optics
With adaptive optics, incoming light that has been distorted by atmospheric fluctuations is instantly corrected by the system. A camera requires high speed and high spatial resolution image acquisition to perform real-time and highly accurate wavefront correction. Furthermore, the wavefront correction is carried out in extremely dark conditions where a laser guide star is measured, necessitating high sensitivity from the camera.
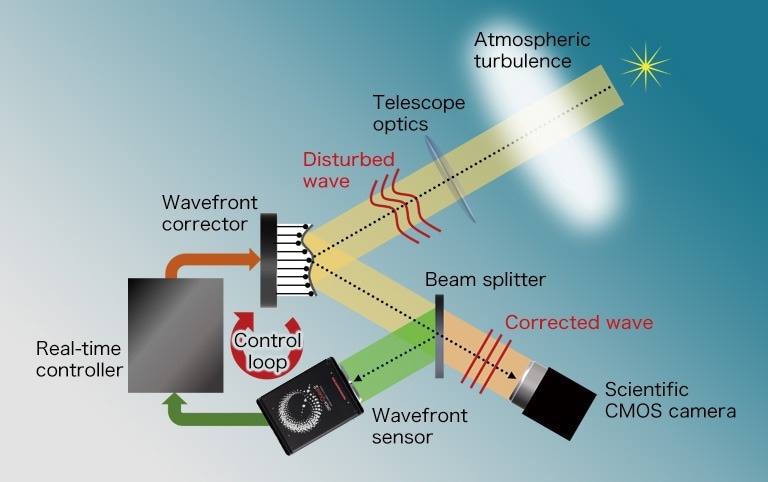
Wavefront correction by adaptive optics. Image Credit: Kodai Yamamoto, Ph.D., Department of Astronomy, Kyoto University
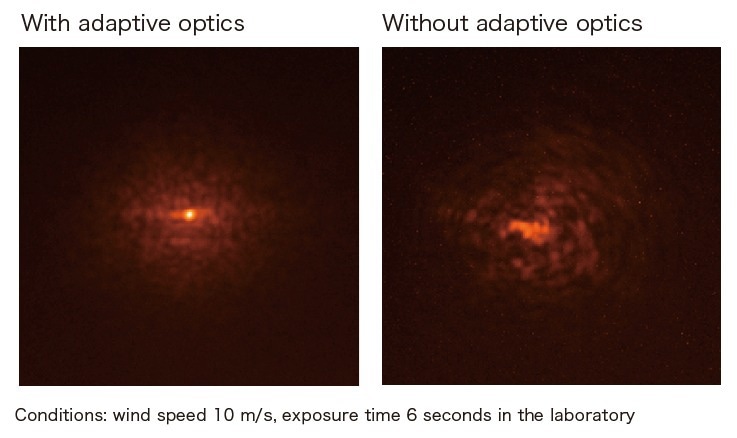
Comparison of Adaptive Optics. Image Credit: Kodai Yamamoto, Ph.D., Department of Astronomy, Kyoto University
HEP / Synchrotron
X-Ray or other high-energy particle imaging is commonly performed using a scientific camera in conjunction with a scintillator. For an imaging system to detect transient phenomena, it must have both high speed and low noise.
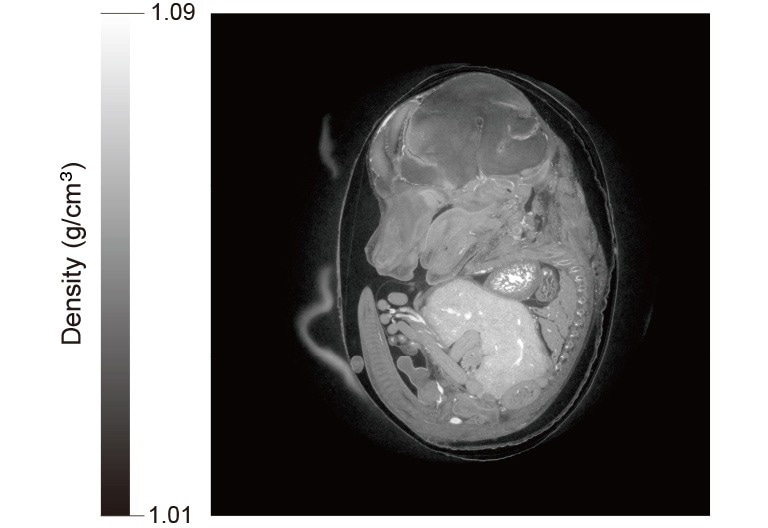
X-Ray phase contrast CT image of mouse embryo. X-Ray phase contrast CT image of mouse embryo from ORCA-Quest combined with High resolution X-Ray imaging system (M11427). Exposure time: 15 milliseconds, Total measurement time: 6.5 minutes. Image Credit: SPring-8 BL20B2 beamline by Dr. Masato Hoshino, Senior researcher at Japan Synchrotron Radiation Research Institute (JASRI)
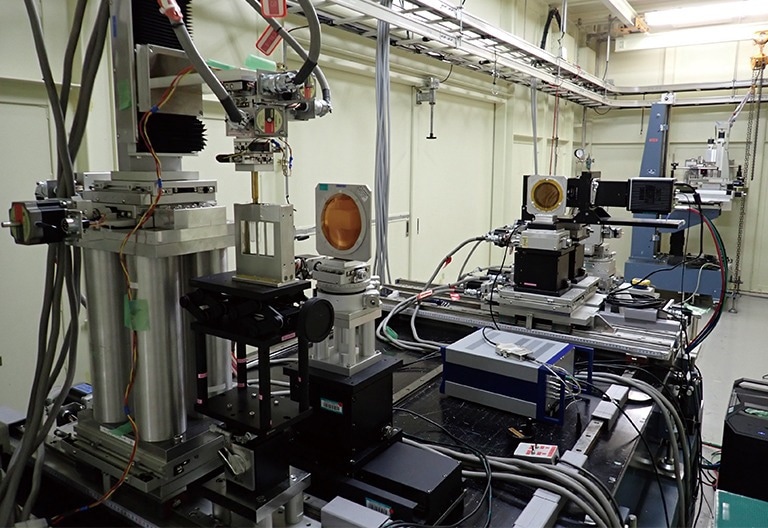
Experimental Setup. Image Credit: SPring-8 BL20B2 beamline by Dr. Masato Hoshino, Senior researcher at Japan Synchrotron Radiation Research Institute (JASRI)
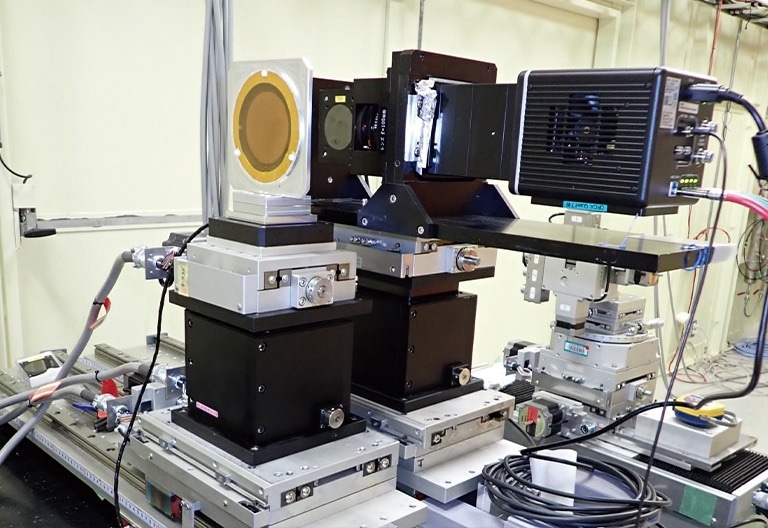
Camera Setup. Image Credit: SPring-8 BL20B2 beamline by Dr. Masato Hoshino, Senior researcher at Japan Synchrotron Radiation Research Institute (JASRI)
Raman Spectroscopy
The Raman effect involves scattering light at a wavelength different from the incident light. By measuring this wavelength shift, Raman spectroscopy utilizes this phenomenon to determine material properties. This technique facilitates structural analysis at the molecular level, offering insights into chemical bonding, crystallinity, and other properties.
Comparison of the Raman spectrum (single frame) in line scan type with equal photon number per pixel Raman microscope.
Raman Image
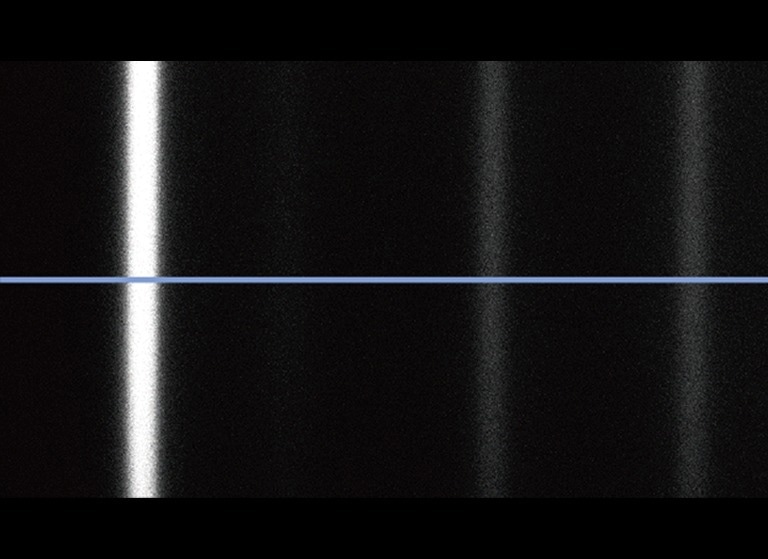
@10 photon/pixel/frame, 532 nm laser excitation. Image Credit: Photon number resolving capability of qCMOS camera for Raman spectroscopy and imaging.
qCMOS
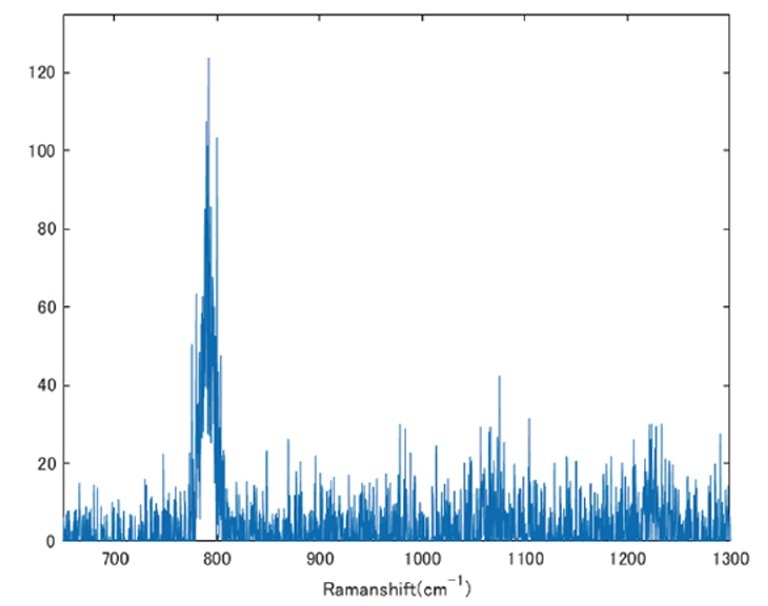
Image Credit: Photon number resolving capability of qCMOS camera for Raman spectroscopy and imaging
EM-CCD
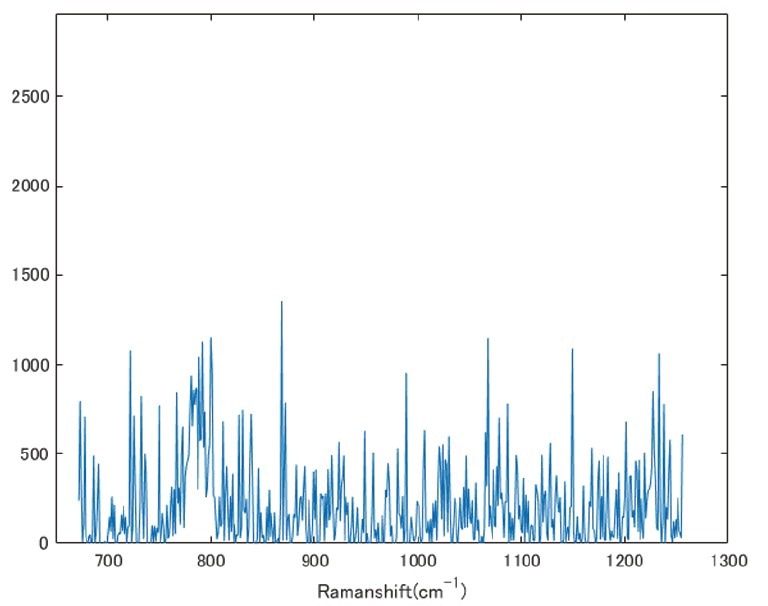
Image Credit: Photon number resolving capability of qCMOS camera for Raman spectroscopy and imaging
Specifications
Source: Hamamatsu Photonics Europe GmbH
. |
. |
Type number |
C15550-22UP |
Imaging device |
qCMOS image sensor |
Effective no. of pixels |
4096 (H) × 2304 (V) |
Cell size |
4.6 μm (H) × 4.6 μm (V) |
Effective area |
18.841 mm (H) × 10.598 mm (V) |
Quantum efficiency |
85 % (peak QE) (typ.) |
Full well capacity |
7000 electrons (typ.) |
Readout speed |
Standard scan*1: 120 frames/s (At full resolution, CoaXPress), 17.6 frames/s (At full resolution, USB)
Ultra quiet scan, PNR, Raw *2: 25.4 frames/s (At full resolution, CoaXPress), 17.6 frames/s (At full resolution, USB) |
Readout noise |
Standard scan: 0.43 electrons rms (typ.), 0.39 electrons median (typ.)
Ultra quiet scan: 0.30 electrons rms (typ.), 0.25 electrons median (typ.) |
Exposure time |
Standard scan*1: 7.2 μs to 1800 s
Ultra quiet scan, PNR, Raw *2: 33.9 μs to 1800 s |
Cooling temperature |
Forced-air cooled (Ambient temperature: +25 °C) : -20 ℃
Water cooled (Water temperature: +25 °C)*3 : -20 ℃
Water cooled (Max cooling; The water temperature is +20 ℃ and the ambient temperature is +20 ℃) *3: -35 ℃ (typ.) |
Dark current |
Forced-air cooled (Ambient temperature: +25 °C) : 0.016 electrons/pixels/s (typ.)
Water cooled (Water temperature: +25 °C) : 0.016 electrons/pixels/s (typ.)
Water cooled (Max cooling; The water temperature is +20 ℃ and the ambient temperature is +20 ℃) : 0.006 electrons/pixels/s (typ.) |
Dynamic range |
23 000 : 1 (rms) (typ.), 28 000 : 1 (median) (typ.)*4 |
External trigger mode |
Edge / Global reset edge / Level / Global reset level / Sync readout / Start |
External trigger signal routing |
SMA |
Trigger delay function |
0 s to 10 s in 1 μs steps |
Trigger output |
Global exposure timing output / Any-row exposure timing output / Trigger ready output / 3 programmable timing outputs / High output / Low output |
External signal output routing |
SMA |
Image processing functions |
Defect pixel correction (ON or OFF, hot pixel correction 3 steps) |
Emulation mode |
Available (ORCA-Quest, ORCA-Fusion) |
Interface |
USB 3.1 Gen 1, CoaXPress (Quad CXP-6) |
A/D converter |
16 bit, 12 bit, 8 bit |
Lens mount |
C-mount*5 |
Power supply |
AC100 V to AC240 V, 50 Hz/60 Hz |
Power consumption |
Approx. 155 VA |
Ambient operating temperature |
0 °C to +40 °C |
Ambient storage temperature |
-10 °C to +50 °C |
Ambient operating humidity |
30 % to 80 % (With no condensation) |
Ambient storage humidity |
90 % Max. (With no condensation) |
*1: Normal area readout mode only
*2: PNR mode and Raw mode can be switched via DCAM configurator. The PNR mode is selected by default.
*3: Water volume is 0.46 L/m.
*4: Calculated from the ratio of the full well capacity and the readout noise in ultra quiet scan
*5: A product for F-mount (C15550-22UP01) is also available. If you wish, please contact your local Hamamatsu representative or distributor. F-mount has a light leakage due to its structure and it might affect your measurements especially with longer exposure time.
Dimensions
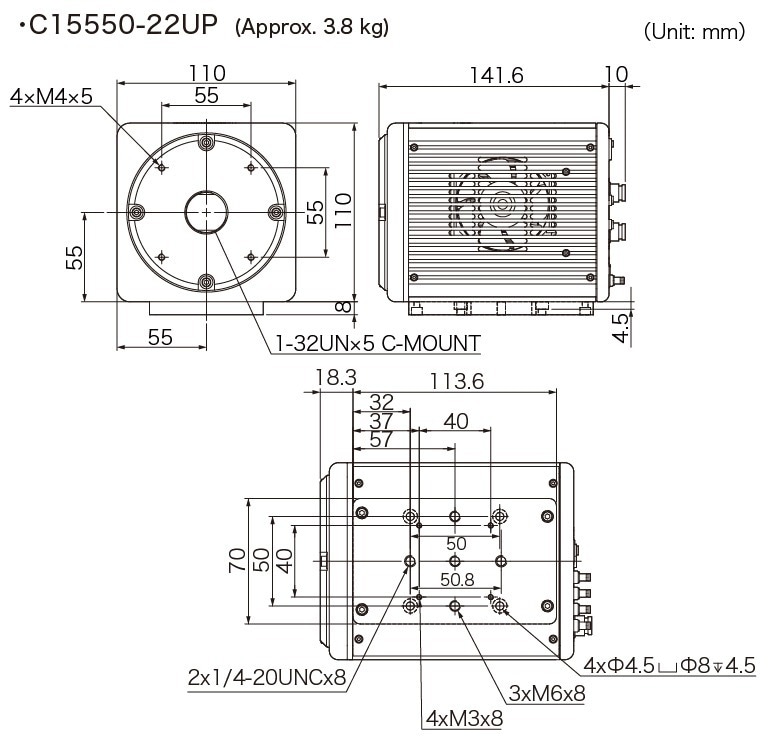
Image Credit: Hamamatsu Photonics Europe GmbH
Software
The software serves as the user interface for all of the features meticulously designed into the cameras, ranging from adjusting exposure to coordinating intricate triggering for experiments involving multiple dimensions.
Camera Simulation Lab
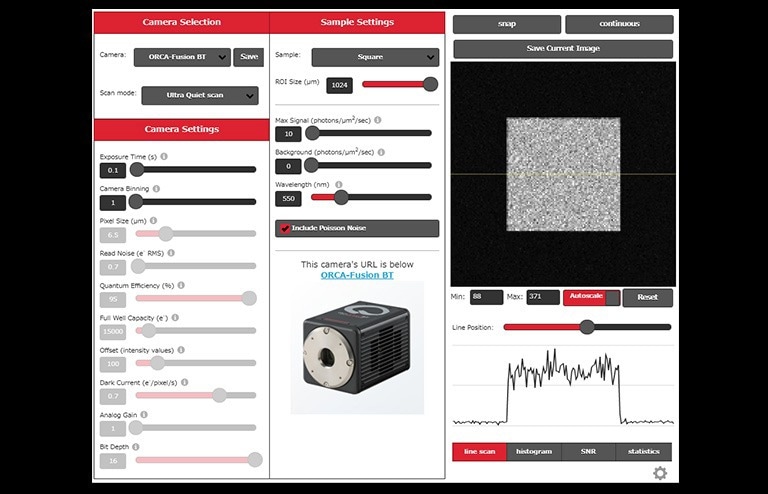
Image Credit: Hamamatsu Photonics Europe GmbH
It is important to choose a camera carefully when using one for industrial or research purposes, considering factors like the object’s wavelength and light intensity. Hamamatsu provides the “Camera simulation lab,” a tool that lets users intuitively examine the simulated images and compare the variations in imaging results caused by camera performance.