Quantitative proteomics is a technique in analytical chemistry that is used to determine the number of proteins in a sample. It is similar to general qualitative proteomics, however, includes a quantifiable component as an additional dimension. The information derived from quantitative proteomics, therefore, yields information about distinguishable physiological characteristics between two different samples as opposed to simply listing the identity of proteins identified in a sample.
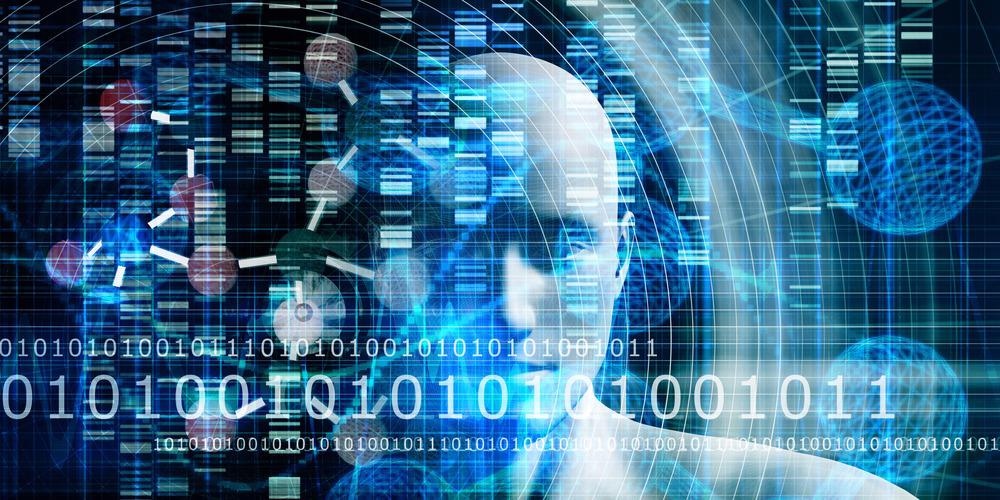
Image Credit: kentoh/Shutterstock.com
the landscape of quantitative proteomics has evolved, beginning in the 1990s with the development of two-dimensional gel electrophoresis and in-gel protein identification using MALDI-TOF. In the early 2000s, gel-based proteomics was largely replaced by liquid chromatography coupled to nanoelectrospray. This produced higher reproducibility and sensitivity. Presently new, single liquid chromatography-mass spectrometry (LC-MS/MS) has improved the resolution of proteomics, enabling the identification of the yeast proteome as well as HeLa cell protein extracts.
What is Quantitative Proteomics Used For?
Quantitative proteomics is a powerful tool in the field of medicine come out as it is principally used to investigate the proteome of disease states, which include cancer. It is also useful in biochemical applications, and mark presents a major subjective study, proteomics is used to identify biomarkers and therapeutic targets.
Over the past several decades, technical advances in mass spectrometry have increased the sensitivity of protein identification and quantification. In addition to this, large-scale identification and resolution of biological mechanisms such as post-translational modifications (notably phosphorylation) have been enabled through quantitative proteomics.
How Is Quantitative Proteomics Performed?
There are two approaches to proteomic analysis. These are top-down proteomics in which whole proteins or large fragments are ionized and subsequently analyzed via mass spectrometry. The second commonly employed approach is bottom-up proteomics; proteins are subject to proteolytic digestion to produce peptides. This technique is more commonly employed due to the 50kDa size limitation present in top-down proteomics.
Samples frequently contain many proteotypic peptides. Subsequently, only a small subset of them can be analyzed in any given mass spectrometer run in addition proteins must be identified in all samples that are tested, therefore the number of proteins available for quantitation is limited. Alongside the limitation imposed by protein abundance, sample complexity also affects the availability of proteins for mass spectrometric quantitation as this is directly proportional to sample complexity.
To reduce sample complexity, methods such as affinity purification are performed. These remove high abundance proteins. Alongside this inline liquid chromatography is also a common technique used before mass spectrometry; this uses a chemical basis for separating peptides to further reduce sample complexity.
Tandem mass spectrometry is a more commonly employed mass spectrometry technique to quantitate selected peptides (MS/MS). The first round of mass spectrometry called MS1 produces a precursor ion spectrum that represents all ionized peptides in the sample. During the second round of mass spectrometry, MS2, individual ions undergo collision-induced fragmentation to produce a fragment ion spectrum for each precursor ion. What results are fragmented spectra, and these are compared to peptide databases to enable specific peptide sequences to be assigned. After assignment, these fragments are computationally organized to produce the predicted protein sequence.
Discovery and Targeted Proteomics
Proteomics research is divided into 2 categories: discovery and targeted proteomics. Discovery proteomics limit the number of samples analyzed to optimize protein identification. In contrast, targeted proteomics limits the number of characteristics that will be surveyed and then optimizes the chromatography, instrument tuning, and the means of acquisition to produce a high throughput with greater sensitivity for hundreds or thousands of samples.
For this reason, discovery proteomics is used to identify a large number of proteins across the broad dynamic range. Targeted quantitative proteomics, by contrast, quantify less than 100 proteins with very high precision, specificity, throughput, and sensitivity.
Absolute and Relative Quantification
There are two types of quantitation relative and absolute. Relative quantitation, as the name suggests, compares individual peptides in a sample to one of an identical, but experimentally modified sample. This is performed in label-free quantitation methods. For relative quantitation methods that do use labels, the process entails labeling proteins from two experimental samples with either isotopically heavy or light atoms which render the peptides in these two samples isotopologs; that differs only in their isotope composition.
Both labeled isotopologues are detected simultaneously during mass spectrometry analysis. The intensities of the peaks derived from the heavy and light peptides are then compared to determine the change in abundance in one sample relative to the other.
Absolute proteomic quantitation uses isotopically labeled peptides whose concentrations are known. The samples are loaded into the test sample and then LC-MS/MS is performed. As with relative quantitation, both the sample and the test reference are analyzed simultaneously. The abundance of the target peptide in the experimental sample is compared to the heavy peptide and back-calculated to determine the initial concentration of the standard using a standard curve to identify the absolute quantitation of the target peptide.
Label-Free Quantitation
Label-free quantitation label-free methods are available for both relative and absolute quantitation. These are best suited for large-scale sample analysis for example in clinical screening or biomarker discovery. They are suited to measuring large changes in protein expression, however, are less reliable for measuring small changes with a limited range of linear quantitative measurement.
In label-free relative quantitation, ion peak intensity is generated only by using LC-MS instead of LC-MS/MS. The signal intensity for all ions is recorded and because they correlate with iron concentration, the relative peptide quantities can be determined directly.
Label-free methods can also be applied to absolute protein quantitation. There are several methods available to do this; exponentially modified protein abundance index (emPAI) estimates protein levels based on the number of peptides detected on the number of theoretically observed tryptic peptides; this is used to approximate absolute abundance in large scale analysis. Absolute protein expression (APEX) uses the number of peptides observed to calculate protein abundance by using correction factors to make the two proportional to one another.
Quantitative proteomics is essential across money spares, most notably when assessing diagnostic expression patterns last unknown to be specific to pathological states. This enables researchers to identify the genes and gene products that are either responsible for or correlate with, a specific state of health.
In the practical setting, it has been used to quantify tumor proteomes and determine the mechanisms of action for drugs. It is also applied more widely to discover disease biomarkers as well as provide insights into biological pathways. Subsequently, it holds great potential for the discovery of diagnostic or prognostic proteins which can be targeted by new therapeutic strategies.
Sources:
- Zhu W, Smith JW, Huang CM. (2010) Mass spectrometry-based label-free quantitative proteomics. J Biomed Biotechnol. doi: 10.1155/2010/840518.
- Old WM, Meyer-Arendt K, Aveline-Wolf L, et al (2005) Comparison of label-free methods for quantifying human proteins by shotgun proteomics. Mol Cell Proteomics. doi: 10.1074/mcp.M500084-MCP200.
- Ahrens CH, Brunner E, Qeli E, et al. (2010) Generating and navigating proteome maps using mass spectrometry. Nat Rev Mol Cell Biol. doi: 10.1038/nrm2973.
- Prakash A, Piening B, Whiteaker J, et al. (2017) Assessing bias in experiment design for large scale mass spectrometry-based quantitative proteomics. Mol Cell Proteomics.. doi: 10.1074/mcp.M600470-MCP200.
Further Reading