Genomics involves the study of the genome, with the location of every gene being mapped and studied within the Human Genome Project. This is significant as the genome and genome mapping study provided comprehensive insight into genes of interest associated with mutations and disease onset. The collection of this data can lead to novel biological targets being explored for research into novel treatments.
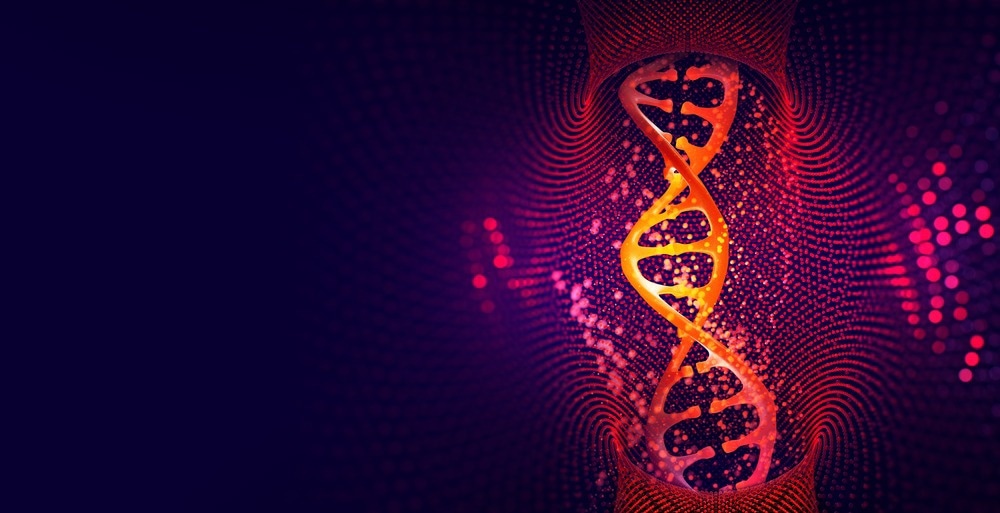
Image Credit: Yurchanka Siarhei/Shutterstock.com
Similarly, proteomics includes the study of the proteome comprising the entire set of proteins produced by cell types. With proteins being the final product of genes, they hold significant functions in the body, comprising regulatory molecules such as hormones and transport proteins, including hemoglobin that helps carry oxygen to organs, antibodies, and more.
The study of the proteome, such as through the novel Human Proteoform Project, aims to comprehensively analyze proteoforms to fully understand the role of proteins in the human body, including their association with many diseases.
Benefits of Combining Genomics and Proteomics
Combining both genomics and proteomics is a significant benefit for many reasons, as this comprehensive approach can cover all disease associations within the body and reveal many biological targets for drug development.
Interestingly, while all the cells within a multicellular organism consist of the same set of genes, this is not true of proteins. Gene expression within a cell can vary due to the type of cell, and variations in gene expression result in different proteins being produced by each cell, subsequently leading to cells having different functions.
Protein variation occurs during the translation process where nucleotide bases are translated into amino acids, which form an array of polypeptide sequences that contribute to the protein's unique structure. There are also other processes that cause modification of proteins after translation, which further differentiate proteins from each other.
The study of both the blueprint of cells and the progression of cells to their functions can aid in understanding the genetic basis of all diseases. The most significant disease studied with proteomic tools includes cancer, and with these approaches being used to identify various protein markers with different expressions, screening, and early disease detection have been improved.
Examples of protein biomarkers used for diseases include CA-125, a cancer biomarker identified in ovarian cancer patients, and PSA, a type of protein that can be used to detect prostate cancer in men.
Whole genome, whole exome, and whole transcriptome sequencing can be carried out for genetic diagnostics for an array of diseases, including rare diseases, as these tests sequence the whole genome, exome, which includes the coding regions of the DNA and the transcriptome, which comprises both coding and non-coding RNA.
These comprehensive testing tools can be used for furthering personalized or precision medicine, which is a medical care approach that assists in choosing the most effective treatment option for a patient based on their heterogeneous disease.
Genomics and proteomics can also aid with preventive medicine, with patients that are more likely to develop a disease due to heritability or the presence of environmentally-borne mutations being provided with preventative steps before onset. This can improve quality of life and alleviate the global disease burden, with diseases being targeted early without the individual's health being affected.
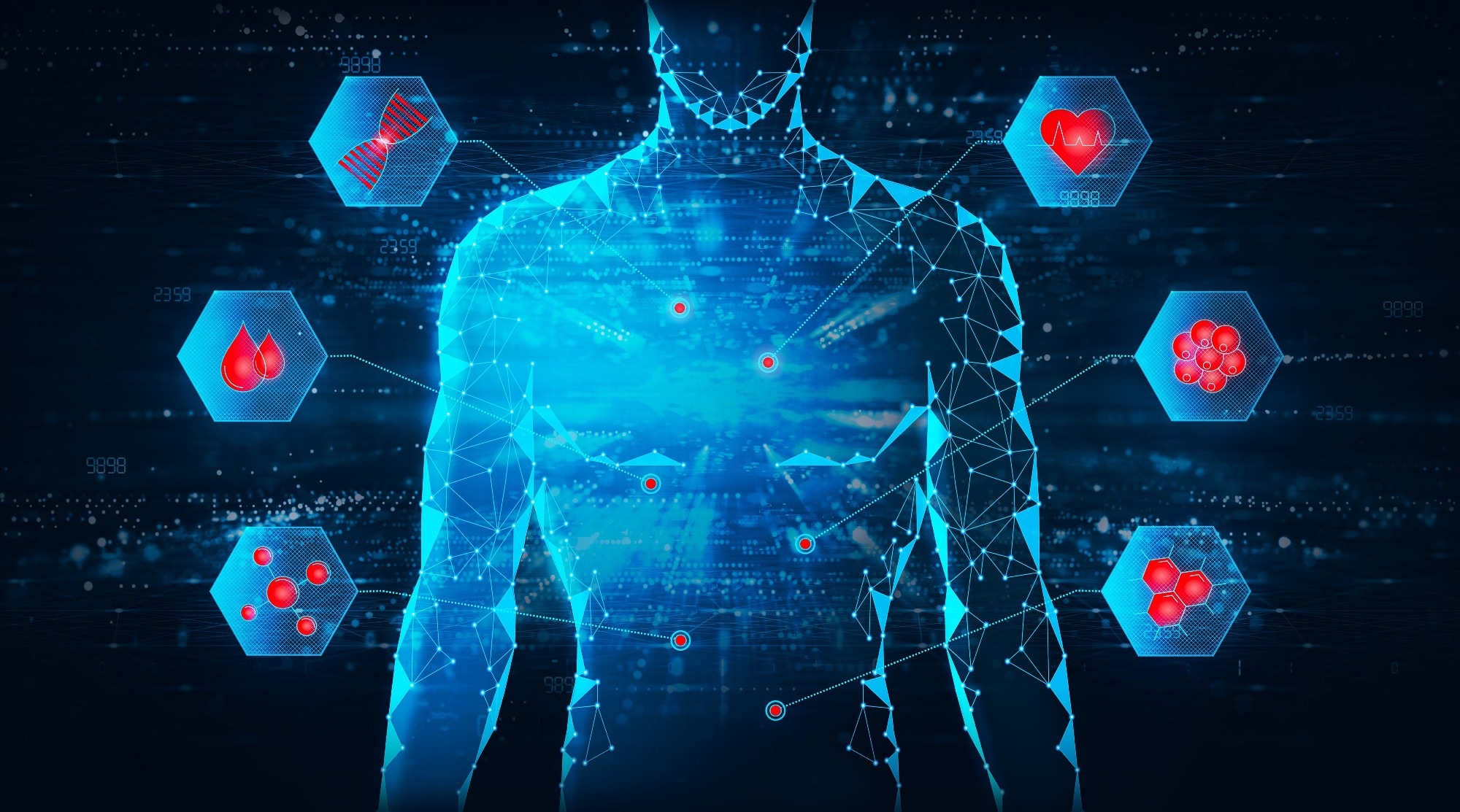
Image Credit: ArtemisDiana/Shutterstock.com
Challenges
While both genomics and proteomics can significantly affect disease control, there are still challenges that prevent the uptake of these approaches to being used globally as a personalized medicine strategy.
The Human Proteoform Project aims to map all the proteoforms within the human body to understand how proteins affect diseases and disorders. However, studying proteins can be difficult because proteins are more difficult to work with than DNA and RNA. The secondary and tertiary structures of proteins require maintenance while being analyzed, and this can be difficult for researchers due to the instability of proteins, including their ability to denature through enzymes, heat, light, and even aggressive mixing.
Additionally, proteins cannot be amplified in the same manner as DNA, and this can make analysis of a small volume difficult, with less abundant species being detected.
Cost is also a major obstacle that has prevented the widespread use of proteomics within laboratories due to requiring complex instruments, technologies, computing power, and consumables.
Future Outlook
The combination of proteomics with genomics is complementary to the information already being explored by genomic mapping, and this can aid in further understanding the human body and its biological elements. Research into these regions can lead to novel biological targets for various diseases, including cancer, neurodegenerative diseases, and even rare diseases.
However, while this combination may significantly affect diagnostics, prognostics, and even treatment in the future, some challenges still require overcoming.
Sources:
- Cho WCS. Proteomics Technologies and challenges. Genomics, Proteomics & Bioinformatics. 2007;5(2):77-85. doi:10.1016/s1672-0229(07)60018-7.
- Curry PD, Broda KL, Carroll CJ. The role of RNA-sequencing as a new genetic diagnosis tool. Current Genetic Medicine Reports. 2021;9(2):13-21. doi:10.1007/s40142-021-00199-x.
- Kopajtich R, Smirnov D, Stenton SL, et al. Integration of proteomics with genomics and transcriptomics increases the diagnostic rate of Mendelian Disorders. 2021. doi:10.1101/2021.03.09.21253187.
Further Reading