In the field of New-Ethology, model organisms like murine models or old-world monkeys are being used to acquire a deeper understanding of the brain's biological processes. Their brain functions mimic those of human beings. They accomplish learned tasks well, making them suitable for behavioral and cognition studies.
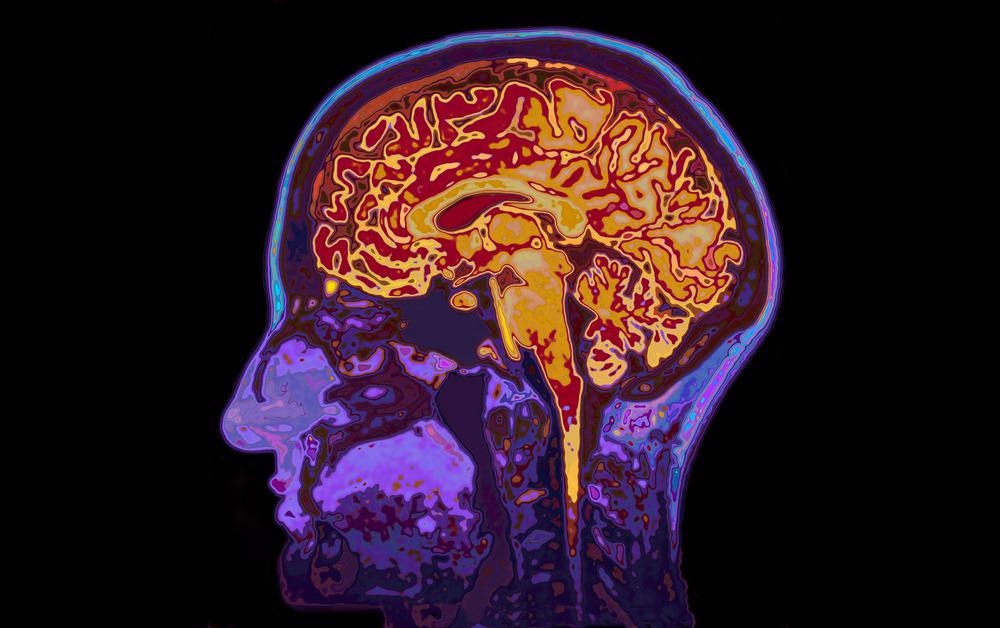
Image Credit: SpeedKingz/Shutterstock.com
Through fluorescence imaging, we now possess the ability to engineer markers that alter their brightness in response to brain activity. Using these sophisticated microscopes, we are now able to visualize motor function at the neural circuit level.
How Two-Photon Microscopy can be Employed for In Vivo Imaging
Videos and images can be viewed in single-cell resolution. These pictures of the brain are being executed on smaller murine models. This is made possible using two-photon microscopy, exciting these fluorophores in a single event. This apparatus is superior to traditional confocal microscopy, on account of its reduced scatter, and its ability to penetrate deep within the tissue. Though this red/infrared light equates to higher energy, penetrating up to half a millimeter below the surface, it is a relatively non-invasive system- as the device is mounted outside the brain.
The light employed has a point spread function, with a radial direction of 0.3 µm, and an axial direction of 0.9 µm. The zones directly above and below this focal point are photon deficient and unable to achieve two-photon excitation. Fluorescence is not possible in these regions and therefore, the only readable signal is found in the region of focus. This focus is directed towards the tissue, where the light will scatter and then refract. This modified light is subsequently captured and quantified by a photomultiplier tube- detecting light using an electron-emitting photocathode upon photon absorption events.
Superlative Nature of Three-Photon Microscopy
Two-photon microscopies have shed new light on live-cell imaging. The near-infrared light we see in use is helping broaden the range of topography and tomography approaches. Yet, many cortical layers, as well as the whole cerebral cortex, had been left unilluminated for some time.
Furthermore, much of the signal is focused on a diffraction-limited region. This is where three-photon microscopy comes into play, resolving structures and neuronal structures with minimal scattering and out-of-focus excitation.
Firstly, this uses a longer wavelength, with a short enough frequency to prevent denaturation, and heating of the sample. It’s been found that the preferred window of wavelength is between 1300 and 1700 nanometers. The 3d confinement (how sharp of signal/peak you get), is much better when performing three-photon excitation. This difference is not quite visible to the naked eye, but when employing this technique to brain tissue imaging, the geometric focus is improved and the severity of the rate of signal decay as a function of imaging depth is lesser.
Using these longer wavelengths while performing three-photon excitation is essential, mostly on account of the fluorophores that must be used in this methodology. The fluorescence emission goes hand in hand with the typical fluorophores used in spectroscopy.
Spectroscopy performed at 1300nm work well with GFP, GCaMP, and other blue/green fluorophores. On the opposite end of the visible spectrum mCherry, mKO/mOrange can be used in tandem with three-photon excitation, yielding exceptional efficacy.
Though longer wavelengths equate to less energy, a three-photon excited signal can actually be stronger than its two-photon counterpart, depending on the region of the assay. Two-photon signals are much brighter when used on the surface, or just below it. What is interesting is, the deeper one delves into white matter, the brighter these longer wavelengths will appear in the final reading, increasing the ratio of pulse energy/depth exponentially.
Once the focal point reaches roughly 700-800 microns deep, the pulse energy required to achieve the same signal is the same for two and three-photon microscopies. After this region, once the sub-cortex is reached, three-photon microscopes will yield a better resolution and more candid results overall.
Applications of Three-Photon Microscopy
Instruments such as these are now being mounted on live murine models, using red light to map out neurons through a 5-gram fiberscope. By fixing these microscopies onto free-moving mice, we can now shed new light on more complex behaviors such as spatial navigation or sleep. In addition, the metabolism and thermodynamics of live bodies can be assayed in greater detail, a noninvasive tool that is seeing new applications in clinical settings.
Brain vasculature can be captured with much less noise, and a more prominent signal when stained with fluorescein dextran. In addition, the 3D reconstruction of entire neurons can be achieved, going as deep as the cerebellum and hippocampus. The cerebellum and hippocampus are infamous for being difficult to image. The cerebellum is 1.25 mm deep, while the hippocampus is surrounded by white matter, which encompasses a scatter activity that is 2.5 times higher than that of grey matter. These deeper images can be acquired if the skin is peeled back while leaving the mouse skull intact. Using the longer wavelengths of 1500-1700nm, GFP fluorescent neurons can be viewed, scoping 100 microns into the brain.
These techniques are under constant modification, as unique developments and apparatuses arise. In the last year alone, RCM-NIR microscope modules have been developed with greater sensitivity to near-infrared radiation, Stroboscopic Ultra-Fast Electron Microscopy have been developed to shorten incoming dc electron pulses to pico/sub-picoseconds, and so on.
Sources:
- Klioutchnikov A, Wallace DJ, Frosz MH, Zeltner R, Sawinski J, Pawlak V, Voit KM, Russell PSJ, Kerr JND. Three-photon head-mounted microscope for imaging deep cortical layers in freely moving rats. Nat Methods. 2020 May;17(5):509-513. doi: 10.1038/s41592-020-0817-9. Epub 2020 May 4. PMID: 32371979.
- Yang, D. S., Mohammed, O. F., & Zewail, A. H. (2010). Scanning ultrafast electron microscopy. Proceedings of the National Academy of Sciences of the United States of America, 107(34), 14993–14998
- Wang, T., Ouzounov, D. G., Wu, C., Horton, N. G., Zhang, B., Wu, C. H., Zhang, Y., Schnitzer, M. J., & Xu, C. (2018). Three-photon imaging of mouse brain structure and function through the intact skull. Nature methods, 15(10), 789–792. https://doi.org/10.1038/s41592-018-0115-y
- Hillman E. M. (2007). Optical brain imaging in vivo: techniques and applications from animal to man. Journal of biomedical optics, 12(5), 051402
- Qin, Z., et al. (2020) Adaptive optics two-photon microscopy enables near-diffraction-limited and functional retinal imaging in vivo. Light: Science & Applications. doi.org/10.1038/s41377-020-0317-9.
- Cheng H, Tong S, Deng X, Li J, Qiu P, Wang K. (2020) In vivo deep-brain imaging of microglia enabled by three-photon fluorescence microscopy. Opt Lett. Sep 15;45(18):5271-5274.
- Tong S, Liu H, Cheng H, He C, Du Y, Zhuang Z, Qiu P, Wang K. (2019)Deep-brain three-photon microscopy excited at 1600 nm with silicone oil immersion. J Biophotonics. (6):e201800423.
Further Reading