The study of plants is vital for gaining a deeper understanding of biological mechanisms and providing knowledge of crop improvement and safety, particularly regarding the global economy.1
Traditionally, plant samples were examined as thin dyed sections, but the current method for researching plant samples is 3D in situ live imaging.
Recently, the study of in situ 3D morphogenesis via fluorescence microscopy has been advanced through the availability of a broad variety of fluorescent tissue-specific reporter lines and genetically encoded biosensors.1,2
Working with 3D plant tissue provides the significant challenge of tissue frequently being inhomogeneous. Further complexities arise with cell walls and chlorophyll-containing tissues absorbing light, restricting the microscopy methods employed.3
Plants also have an inherent geometry with aerial sections that have natural tropisms for light and roots affected by gravity. As a result, the sample setup must satisfy physiological conditions, such as temperature or light, and physical conditions, such as large sample size and vertical mounting.4
From an imaging perspective, fluorescence microscopy introduces obstacles due to lasers emitting photons at several orders of magnitude higher than the sunlight plants are usually exposed to.5
This may affect photosynthesis, light exposure, and local temperature, which can all result in a sample deteriorating or the imaging of artifacts.1,4 A sophisticated solution to these challenges is using light-sheet fluorescence microscopy (LSFM).
LSFM utilizes a sheet of light for selective plane excitation while information is collected simultaneously using an uncoupled emission objective and a camera.6 This setup enables large samples to be examined as the beam paths can penetrate the tissue.
Only a thin sheet is illuminated, which results in low phototoxicity. Most LSFM setups enable vertical sample embedding and complete environmental control, which is fundamental for sample health.
This article discusses the challenges associated with using microscopy to image plants, considering LSFM and data processing, and how Bruker Luxendo can help overcome these barriers and produce meaningful data.
Challenges of Plant Imaging
Capturing Dynamic Processes
Most biological processes are intrinsically dynamic. This results in the acquisition of data over time frequently being preferred to single timepoint acquisition that provides only a snapshot of information.
However, live imaging is a trade-off between sample preservation and acquiring good data with adequate signal quality and resolution.4
Visualizing Tissues
Although there is growing availability of tissue-specific fluorescent markers, dyes are still frequently utilized for visualizing tissues of interest. However, dyes may be bleached by exposure to the laser, with some potentially becoming toxic.2 This is why reducing photons with methods such as LSFM is essential for sample health.
Aerial Sections vs Roots
Studying plants outside the sprouting phase means that aerial sections and roots must be considered. Aerial sections contain chlorophyll which reacts to light exposure and scatters light. The cells containing chlorophyll, namely chloroplasts, are autofluorescent and emit additional fluorescence.1
Roots, particularly root tips, are commonly studied in morphogenesis, but they strongly react to gravity, and growth geometry can rapidly change. This results in the need for adaptive microscopy approaches to enable time-lapses greater than 30 minutes.2
Light-sheet fluorescence microscopes have various components that make them ideal for overcoming these considerations. Voluminous sample chambers offer space for sample growth, while vertical embedding considers gravitation and environmental control produces consistent sample conditions.
To enhance the quality of the data, images can be acquired from multiple views (e.g., Bruker’s MuVi SPIM) and merged to obtain data with high signal and resolution. To overcome any sample movement and growth outside the desired field of view (FOV), adaptive autofocus aids in automatic sample tracking.
Cell Walls
Like an exoskeleton, cell walls offer chemical and mechanical resistance to plants. They comprise 40%-60% cellulose, 10%-to-24% lignin, and varying quantities of other cell wall proteins. These components result in a complex 3D network where cellulose fibrils may further trigger light scattering.7
However, as cell walls have a 3D geometry, the ability to image living plants in situ significantly helps to resolve sample preparation challenges, including plant section quality or identifying the proper position and FOV of interest in a section.7
Challenges of LSFM
Light Interaction with Matter
Light interacts with matter, causing light ray interference via transmission, refraction, reflection, absorption, diffraction, and scattering changes.8,9 The effect of matter on light rays can be especially evident when there is mismatching of refractive indices of the sample, embedding medium, and materials.
The optical properties of the system may be optimized by employing a low numerical aperture (NA) illumination objective and a high NA detection objective to facilitate a thin light-sheet with wide-field detection.5
Artifacts that include striping and shadowing are particularly prominent in static single-side illumination setups. A pivoted light sheet and multi-view approach can minimize these issues. Lattice LSFM, in conjunction with structured illumination methods, can further enhance data resolution.10
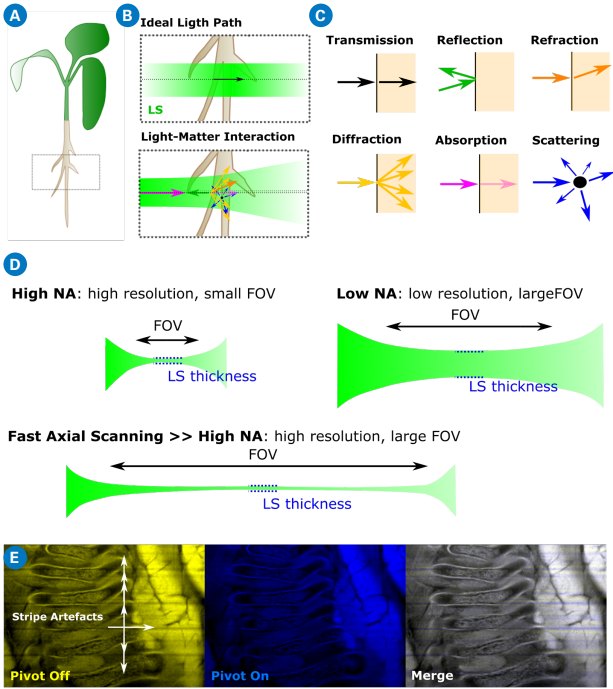
Figure 1. Light interacts with matter. (A) Overview of imaged plant. (B) Ideal light path versus the light path encountered due to light-matter interaction. (C) Breakdown of potential light-matter interactions. (D) The light-sheet setup impacts resolution and FOV. (E) Using a pivoted LS helps to address artifacts such as striping. In this case it shows intestine tissue, but the same principles apply for plant tissues. Panel (B) and (C) adapted from9. Image Credit: Bruker Nano Surfaces and Metrology
Light-Sheet Thickness
The light sheet is central to everything in LSFM. The amount of sample that is illuminated is determined by the sheet thickness. Additionally, the thinner the sheet, the higher the resolution in z. The entire FOV is homogenously illuminated when used with fast axial scanning.
The geometry of the beam is also essential, especially concerning the placement of the beam waist in the center of the FOV.11
The MuVi SPIM generates a homogenously illuminated FOV via fast axial scanning by sweeping a tightly focused Gaussian beam over the illumination axis. This results in an elongated, uniform, and thin light sheet that offers uniform axial resolution over large FOVs.
Multi-View Imaging
Data may be obtained from multiple angles and views and then fused to improve the quality of the data as well as minimize artifacts. The fusion can be conducted with or without fiduciary markers. This provides the ability to cover large specimens with high-quality images and isotropic spatial resolution.12
The MuVi SPIM facilitates sample rotation which allows a 360° view. This makes it the fastest multi-angle view system currently on the market.
The next-generation MuVi SPIM offers an unmatched signal-to-noise ratio because of adjustable light-sheet thickness, light-sheet scanning, robust aberration tolerance, and dual de-striping by pivot scanning.
Flexibility in Setup and Embedding
Most LSFM systems facilitate vertical sample embedding and sample chamber access. Multiple embedding options exist depending on what stage and organ of the plant is being studied.
For example, a jellifying media, such as phytogel or low-melting agarose, can be used with holders, such as glass capillary or FEP tubes, to create a top-holding or bottom-holding setup.3
Environmental control can be formed in the sample chamber and remain stable over time. The sample chamber can also be filled with medium, which may be exchanged over time to prevent the wearing-off or building-up of compounds using motorized pumps.
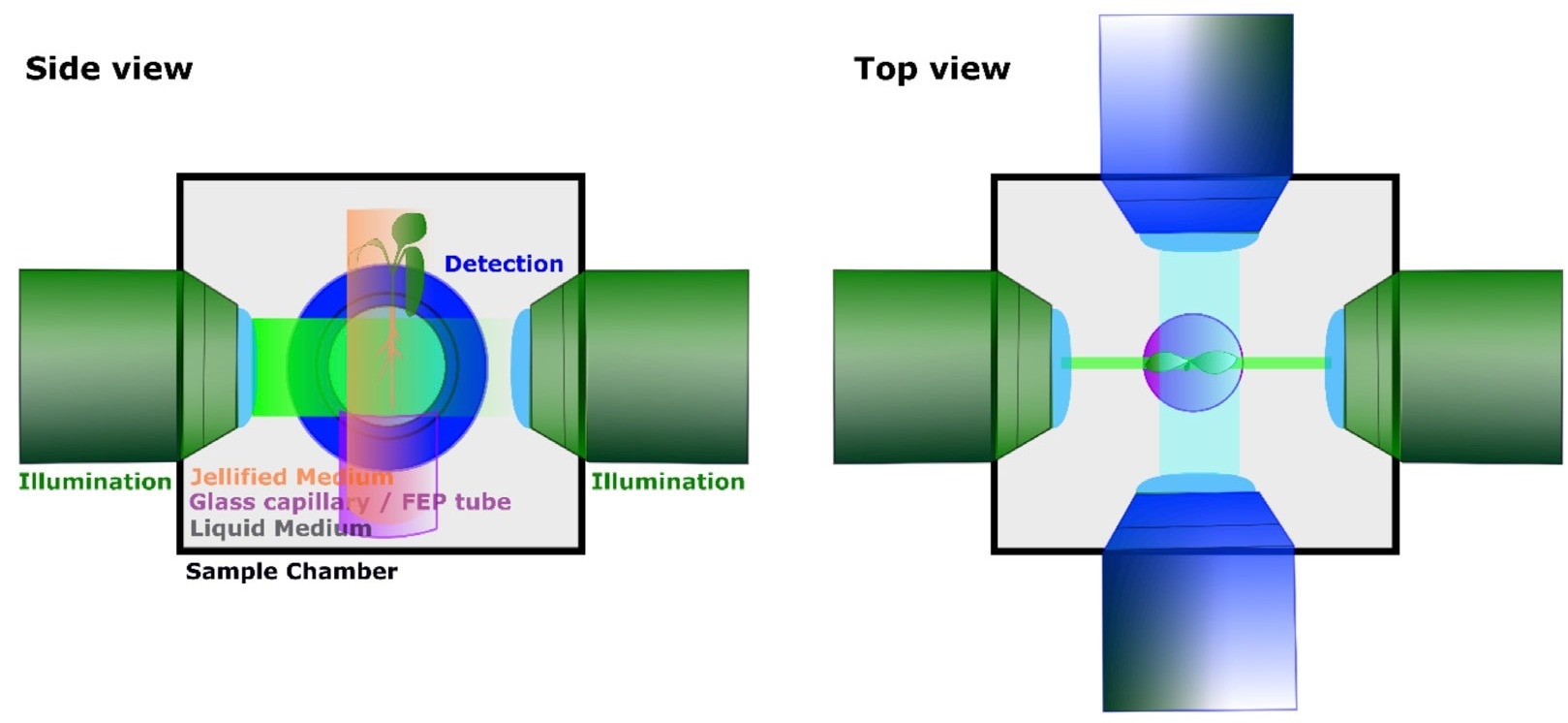
Figure 2. Overview of MuVi SPIM setup where plant roots are imaged. The sample is embedded in a jellifying medium with a glass capillary or FEP tube. The top view on the right shows the optical setup with two illumination objectives (green) and two emission objectives (blue). Image Credit: Bruker Nano Surfaces and Metrology
Applying more advanced imaging techniques or transient perturbation of the system is often valuable to observe biological behavior and provide novel insights into processes' biology.4
Bruker Luxendo offers solutions to achieve this, including light-sheet solutions for photomanipulation (PM module) and advanced imaging (AIM – advanced imaging module) methods.
Data Challenge
Handling Data Analysis with Luxendo Software
A significant challenge in the current data era is analyzing and storing acquired data, particularly LSFM, where datasets may be in the terabyte range.13 The LuxBundle from Bruker enables effective image registration and fusion, providing data processing with application-specific software solutions.
Luxendo Bruker also offers the well-known ACQUIFER HIVE solution to ensure the long-term storage and archiving of data.
Application-specific programs are developing to assist image plant cell segmentation and analysis, such as lineage tracing (e.g., MorphographX,14 PlantSeg,15 or MARS/ALT16).
Application Example
LSFM to Study ANNEXIN 1 in Arabidopsis
Annexins, an evolutionarily conserved superfamily of multifunctional proteins, are essential for many processes, including cell differentiation, growth, and stress responses.
In this case study, Ticha et al. produced a transgenic Arabidopsis line to visualize endogenous ANNEXIN 1 (ANN1) and examine the in vivo distribution via LSFM.17
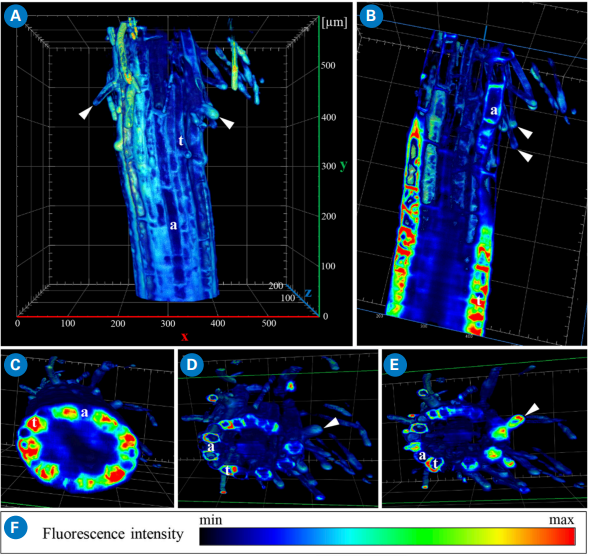
Figure 3. 3D rendering of ANN1-GFP distribution in Arabidopsis. MuVI SPIM obtained data, and after post-processing, 3D reconstruction was made by Arivis. Pseudocolors show fluorescence intensity, with the lowest corresponding to black and the highest fluorescence intensity corresponding to red. Arrowheads point to root hair tips, (t) points trichoblasts, and (a) atrichoblasts. Figure reproduced with permission from Tichá et al.17. Image Credit: Bruker Nano Surfaces and Metrology
Conclusion
Several challenges arise during the imaging of living plants, such as sample properties (e.g. aerial parts and roots, cell walls, chlorophyll), sample-friendly imaging (e.g. embedding, time-lapses, low phototoxicity), tissue visualization (e.g. dyes vs reporter lines), and effective data handling (e.g. processing and storage).
Collaborating with end users and application specialists, Bruker Luxendo has the experience required to deliver expert commercial LSFM solutions that can significantly aid the imaging of living and naturally growing plants.
Acknowledgments
Produced from materials originally authored by Elisabeth Kugler, Monika Löschinger, Malte Wachsmuth, and Melissa Martin at Bruker.
References and Further Reading
- M. Ovecka et al., “Imaging plant cells and organs with light-sheet and super-resolution microscopy,” Plant Physiology, vol. 188, no. 2, pp. 683–702, Feb. 2022, doi: 10.1093/plphys/kiab349.
- L. Colin et al., “Imaging the living plant cell: From probes to quantification,” The Plant Cell, vol. 34, no. 1, pp. 247–272, Jan. 2022, doi: 10.1093/plcell/koab237.
- B. Berthet and A. Maizel, “Light sheet microscopy and live imaging of plants,” Journal of Microscopy, vol. 263, no. 2, pp. 158–164, 2016, doi: 10.1111/jmi.12393.
- G. V. Reddy, S. P. Gordon, and E. M. Meyerowitz, “Unravelling developmental dynamics: transient intervention and live imaging in plants,” Nat Rev Mol Cell Biol, vol. 8, no. 6, Art. no. 6, Jun. 2007, doi: 10.1038/nrm2188.
- E. H. K. Stelzer, “Light-sheet fluorescence microscopy for quantitative biology,” Nat. Methods, vol. 12, no. 1, pp. 23–26, Jan. 2015, doi: 10.1038/nmeth.3219.
- J. Huisken, J. Swoger, F. Del Bene, J. Wittbrodt, and E. H. K. Stelzer, “Optical sectioning deep inside live embryos by selective plane illumination microscopy,” Science, vol. 305, no. 5686, pp. 1007–1009, Aug. 2004, doi: 10.1126/science.1100035.
- G. Costa and I. Plazanet, “Plant Cell Wall, a Challenge for Its Characterisation,” Advances in Biological Chemistry, vol. 6, no. 3, Art. no. 3, May 2016, doi: 10.4236/abc.2016.63008.
- J. Ryan, A. R. Gerhold, V. Boudreau, L. Smith, and P. S. Maddox, “Introduction to Modern Methods in Light Microscopy,” in Light Microscopy: Methods and Protocols, Y. Markaki and H. Harz, Eds. New York, NY: Springer, 2017, pp. 1–15. doi: 10.1007/978-1-4939-6810-7_1.
- E. Kugler and E. Reynaud, “LSFM series – Surfing on the data freak wave! Part II: Before imaging: Know your sample (geometry),” FocalPlane, Oct. 10, 2020. https://focalplane.biologists.com/2020/10/10/lsfm-series-surfing-on-the-data-freak-wave-part-ii-before-imaging-know-your-sample-geometry/ (accessed Nov. 16, 2022).
- P. J. Keller et al., “Fast, high-contrast imaging of animal development with scanned light sheet-based structured-illumination microscopy,” Nat Methods, vol. 7, no. 8, pp. 637–642, Aug. 2010, doi: 10.1038/nmeth.1476.
- M. Weber, M. Mickoleit, and J. Huisken, “Light sheet microscopy,” Methods Cell Biol., vol. 123, pp. 193–215, 2014, doi: 10.1016/B978-0-12-420138-5.00011-2.
- R. K. Chhetri, F. Amat, Y. Wan, B. Höckendorf, W. C. Lemon, and P. J. Keller, “Wholeanimal functional and developmental imaging with isotropic spatial resolution,” Nat Meth, vol. 12, no. 12, pp. 1171–1178, Dec. 2015, doi: 10.1038/nmeth.3632.
- A. Schlaeppi et al., “Meeting in the Middle: Towards Successful Multidisciplinary Bioimage Analysis Collaboration,” Frontiers in Bioinformatics, vol. 2, 2022, Accessed: Apr. 19, 2022. [Online]. Available: https://www.frontiersin.org/article/10.3389/fbinf.2022.889755
- P. Barbier de Reuille et al., “MorphoGraphX: A platform for quantifying morphogenesis in 4D,” eLife, vol. 4, p. e05864, May 2015, doi: 10.7554/eLife.05864.
- A. Wolny et al., “Accurate and versatile 3D segmentation of plant tissues at cellular resolution,” eLife, vol. 9, p. e57613, Jul. 2020, doi: 10.7554/eLife.57613.
- R. Fernandez et al., “Imaging plant growth in 4D: robust tissue reconstruction and lineaging at cell resolution,” Nat Methods, vol. 7, no. 7, Art. no. 7, Jul. 2010, doi: 10.1038/nmeth.1472.
- M. Tichá et al., “Advanced Microscopy Reveals Complex Developmental and Subcellular Localization Patterns of ANNEXIN 1 in Arabidopsis,” PubMed, Accessed: Jan. 10, 2023. [Online]. Available: https://pubmed.ncbi.nlm.nih.gov/32849711/
About Bruker Nano Surfaces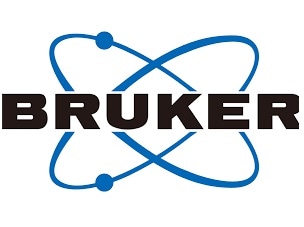
Bruker Nano Surfaces provides high-performance, specialized analysis and testing technology for the widest range of research and production applications.
Our broad portfolio of 2D and 3D surface profiler solutions supply the specific information needed to answer R&D, QA/QC, and surface measurement questions with speed, accuracy, and ease. And our tribometers and mechanical testers deliver practical data used to help improve development of materials and tribological systems. Bruker’s industry-leading quantitative nanomechanical and nanotribological test instruments are specifically designed to enable new frontiers in nanoscale materials characterization, materials development, and process monitoring.
Bruker has been leading the expansion of atomic force microscope (AFM) capabilities since the very beginning, and our systems are the most cited AFMs in the world. Our comprehensive suite of AFMs enable scientists around the world to make discoveries and advance their understanding of materials and biological systems. With our nanoIR technology, Bruker is now also the recognized leader in photothermal IR spectroscopy from the nanoscale to the sub-micron and macro scales. And, as the only AFM manufacturer with a state-of-the-art probes nanofabrication facility and world-wide, application-specific customer support, Bruker is uniquely positioned to provide the equipment, guidance, and support for all your nanoscale research needs.
Bruker’s suite of fluorescence microscopy systems provides a full range of solutions for life science researchers. Our multiphoton imaging systems provide the imaging depth, speed and resolution required for intravital imaging applications, and our confocal systems enable cell biologists to study function and structure using live-cell imaging at speeds and durations previously not possible. Bruker’s super-resolution microscopes are setting new standards with quantitative single molecule localization that allows for the direct investigation of the molecular positions and distribution of proteins within the cellular environment. And our Luxendo light-sheet microscopes, are revolutionizing long-term studies in developmental biology and investigation of dynamic processes in cell culture and small animal models.
In addition to developing and manufacturing next-generation systems to help our customers’ current and future applications, Bruker is also very active in acquiring and partnering with innovative companies to continue to expand our range of enabling technologies and solutions. Recent additions to the Bruker Nano Surfaces family include Alicona Imaging, Anasys Instruments, Hysitron, JPK Instruments, and Luxendo.
Whatever your measurement and analysis needs, whatever your material or scale of investigation, Bruker has a specialized high-performance solution for you.
Territories Serviced
Global
Sponsored Content Policy: News-Medical.net publishes articles and related content that may be derived from sources where we have existing commercial relationships, provided such content adds value to the core editorial ethos of News-Medical.Net which is to educate and inform site visitors interested in medical research, science, medical devices and treatments.