Antibody treatment is one of the most important strategies used to treat multiple ailments. It is important to optimize the development of therapeutic antibodies in the context of their efficacy, safety, and manufacturability. This article discusses the steps associated with optimizing antibody therapeutics.
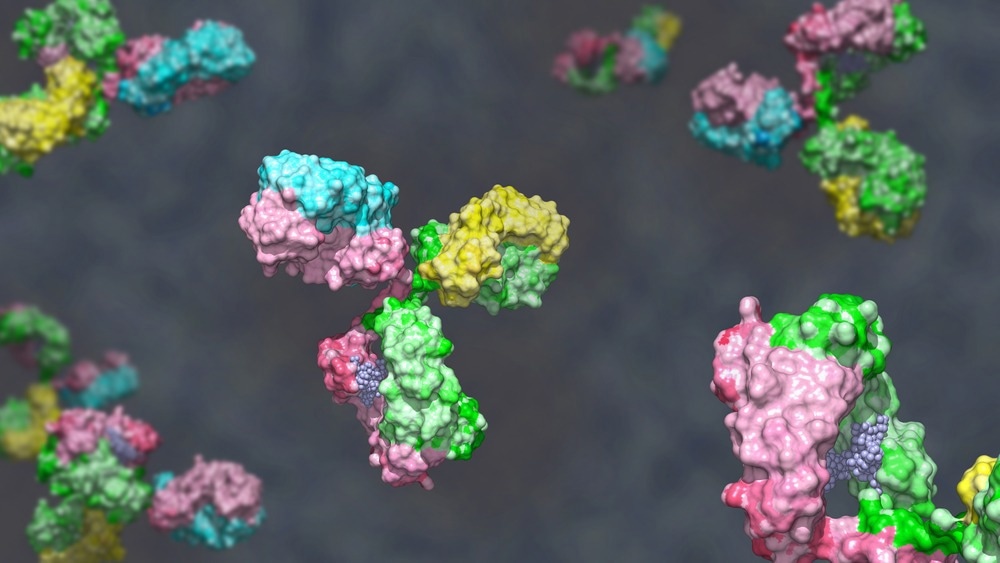
Image Credit: Huen Structure Bio/Shutterstock.com
Optimizing Antibody Therapeutics
One of the primary aspects of antibody optimization is the reduction of a non-human antibody's immunogenicity in humans. This can be achieved via antibody humanization and deimmunization strategies. The following sections focus on the various steps associated with optimizing antibody therapeutics.
Antibody Humanization and Deimmunization
The first strategy used to reduce immunogenicity involves the development of a chimeric antibody, which is carried out by fusing variable regions of a non-human antibody with a human antibody constant region. Additionally, to further reduce immunogenicity, the variable region of the chimeric antibody is modified to increase its level of similarity to naturally occurring antibodies. This technique is referred to as antibody humanization.
Antibody humanization is typically performed by analyzing the difference between non-human antibody sequences and their human homologs. Some common methods associated with antibody humanization are computer-aided design, yeast display, and phage display methods. Two of the most popular software for computer-aided design are BioLuminate and MOE.
Several studies have shown that fully humanized monoclonal antibodies may still exhibit immunogenicity. The key factors that influence the immunogenicity of therapeutic antibodies are epitope sequences of the antibody, dose, and route.
Deimmunization involves identifying and removing major antigenic epitopes (e.g., T cell, B cell, and major histocompatibility complex epitopes). Scientists use computational tools, such as Protean 3D, to identify these epitomes in the query sequences.
Human immunogenicity is also repressed by inducing immune tolerance. This can be achieved by introducing Treg epitopes into the antibody structure, stimulating Treg cell functions, and providing immune tolerance. The immune tolerization technique enables the development of clinically effective antibodies with the less immunogenic property.
Optimization of Antibody Efficacy and Antibody Specificity
Affinity maturation is a method that focuses on improving the binding capacity of antibodies to their target antigen. This is an essential step for optimizing antibody efficacy. Binding antibodies to a specific antigen is a critical function of a therapeutic antibody. Scientists use several methods for antibody affinity maturation, including targeted mutagenesis, random mutagenesis, chain shuffling, and in silico strategies.
Multiple non-covalent interactions occur during antigen-antibody reactions. Scientists reported that they could significantly enhance antigen binding affinity against an antibody via in silico affinity maturation. Researchers incorporate multiple designed mutations in an engineered antibody, which improve the binding efficacy of an antibody against a specific antigen. Computed electrostatics have also significantly contributed to the optimization of antibody efficacy.
Random and targeted mutagenesis are two of the most common strategies associated with antibody specificity optimization. Computational platforms, e.g., BioLuminate, are used for affinity maturation function, which also contributes to the optimization of antibody specificity. Computed electrostatic models showed that higher electrostatics promotes greater binding specificity.
Hydrophobic interactions and a lower frequency of electrostatic interactions cause conformational flexibility in molecules. Besides improving the binding efficacy of an antibody against a specific antigen, the biological efficacy of the antibody can also be altered via Fc engineering.
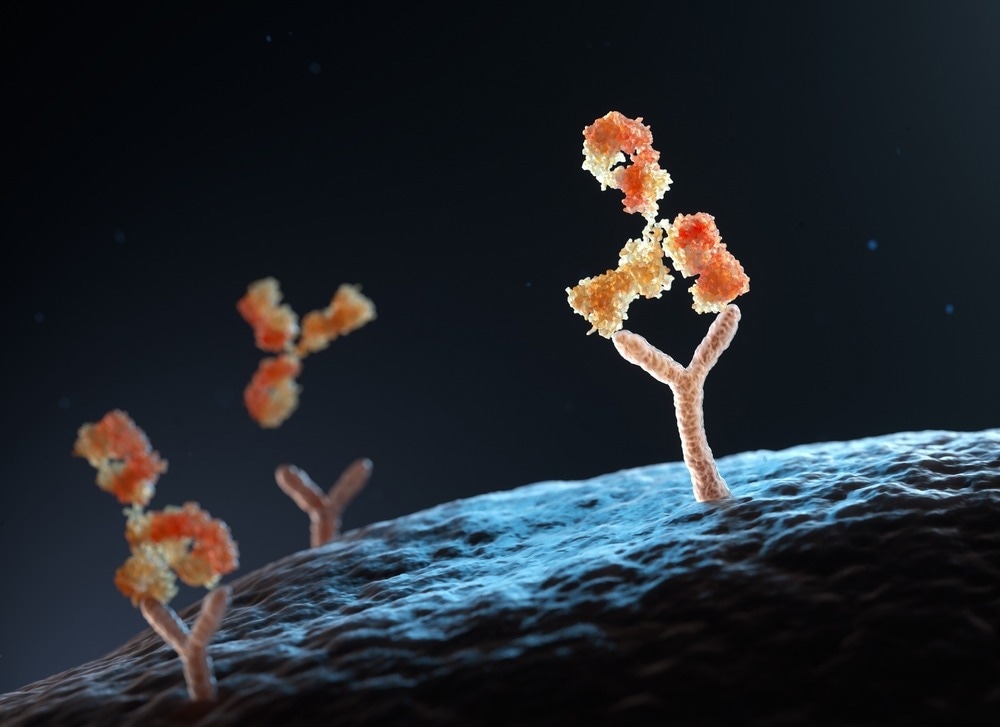
Image Credit: Tatiana Shepeleva/Shutterstock.com
Optimization of Antibody Pharmacokinetic Properties
Some advantages related to pharmacokinetic improvements of a therapeutic antibody are decreased dosage, subcutaneous formulation, and lower cost of production. Additionally, pharmacokinetic improvements help prolong the dosage intervals, which is beneficial for patients.
It is imperative to reduce the non-specific clearance of therapeutic antibodies, which occurs via two key mechanisms. The first mechanism is realized when antibodies are non-specifically internalized into the cellular endosomes, and the second mechanism occurs during antigen-binding-mediated internalization, which leads to clearance.
Scientists have devised several strategies to reduce the non-specific clearance of antibodies, including lowering an antibody's isoelectric point (pI), which improves its half-life. Hence, the primary selection of antibodies from libraries with lower pI or different humanization variants could be an effective way to develop therapeutic antibodies with decreased non-specific clearance rate. Similarly, selecting pH-sensitive antibodies from a library of humanization or antibody variants is an effective strategy for selecting antibodies with reduced non-specific clearance.
Optimization of Antibodies Pharmaceutical Properties
Some of the most desirable properties required of a therapeutic antibody are high solubility, thermostability, low heterogeneity, and considerable chemical stability. These properties enable significant retention of the biological activity of an antibody during storage, improve production efficacy, decrease the cost of production, and facilitate a high quality of large-concentration formulation.
Researchers stated that poor thermostability of therapeutic antibodies could lead to aggregation and low expression. Library-based site mutagenesis is an effective approach to optimizing the thermostability of a potential antibody. Additionally, computer-aided antibody structure modifications are another approach used to optimize antibody thermostability in silico.
Studies have reported that clinical antibodies must exhibit high solubility and very low viscosity. One of the effective methods to improve solubility is the removal of surface hydrophobicity. Optimization of the thermal stability of antibodies is carried out via different processes, including structure-based engineering, i.e., by changing the pI.
Sources:
- S, Karlijn. et al. (2022) Optimization of Anti-SARS-CoV-2 Neutralizing Antibody Therapies: Roadmap to Improve Clinical Effectiveness and Implementation. Frontiers in Medical Technology. https://doi.org/10.3389/fmedt.2022.867982
- Makowski, E.K. et al. (2022) Co-optimization of therapeutic antibody affinity and specificity using machine learning models that generalize to novel mutational space. Nature Communications, 13(3788). https://doi.org/10.1038/s41467-022-31457-3
- Wang, B. et al. (2021) Optimization of therapeutic antibodies. Antibody Therapeutics. 4(1), pp. 45-54. doi: 10.1093/abt/tbab003
- Bauer, J. et al. (2020) Rational optimization of a monoclonal antibody improves the aggregation propensity and enhances the CMC properties along the entire pharmaceutical process chain. mAbs. 12(1). DOI: 10.1080/19420862.2020.1787121
- Lu, R.M. et al. (2020) Development of therapeutic antibodies for the treatment of diseases. Journal of Biomedical Science. 27(1). https://doi.org/10.1186/s12929-019-0592-z
- Warszawski S, et al. (2020) Correction: Optimizing antibody affinity and stability by the automated design of the variable light-heavy chain interfaces. PLOS Computational Biology, 16(10): e1008382. https://doi.org/10.1371/journal.pcbi.1008382 View correction
Further Reading