The industry’s point of view on therapeutic antibodies
Over the past several years, the interest in utilizing monoclonal antibodies (MAbs) for therapeutic purposes has risen significantly. By definition, MAbs are protein molecules that are often produced by hybridoma cells through recombinant deoxyribonucleic acid (DNA) technology. MAbs have been used as stand-alone drugs for the treatment of various diseases and have recently been studied for drug carriers to treat various other diseases including cancer, autoimmune diseases, and neurological disorders.
MAbs are often produced from mammalian cells; however, industries have often faced several challenges when utilizing this manufacturing approach. Some of the factors that contribute to the difficulties in this production method include low yield, medium complexity, serum requirement, and shear sensitivity. In an effort to overcome these challenges, several highly productive cell clones have been developed in order to increase the specific productivity per cell.
Overall, biopharmaceutical companies looking to produce MAbs are interested in maintaining the desired quality attributes of the antibodies while reducing time to market and the costs associated with their production. Furthermore, since most antibody therapies require large doses that are administered to patients over extended periods, manufacturing capacity is of the highest priority to meet these clinical requirements.
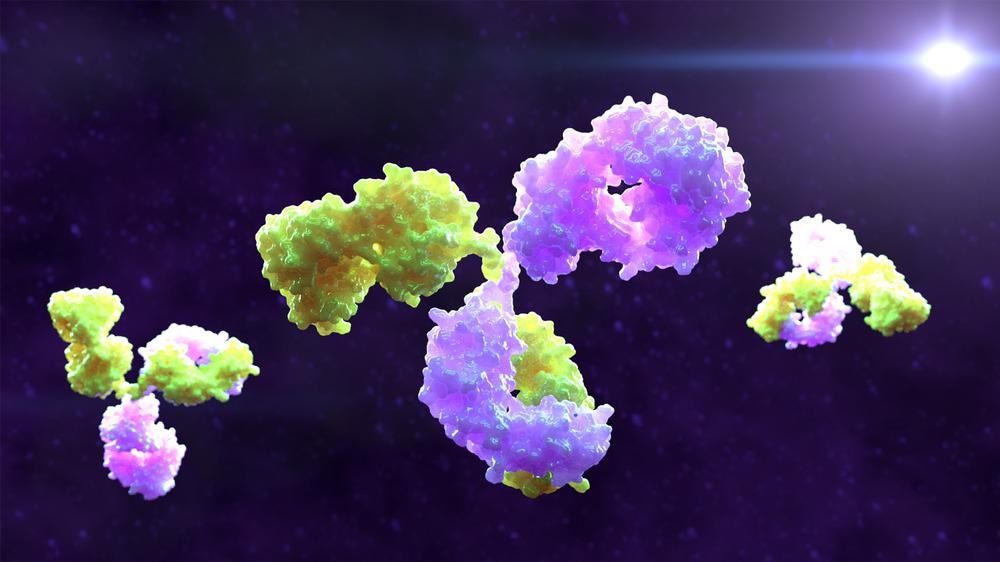
Image Credit: Alpha Tauri 3D Graphics/Shutterstock.com
A brief history of the CHO cell line
In 1956, Theodore Puck first isolated the Chinese Hamster Ovary (CHO) cell line, which was soon followed by additional developments that allowed for the development of subclones with specific mutations that allow for more efficient protein production. For example, CHO DXB11, as well as CHODG44 and CHO-GS-/- hosts have been generated through the incorporation of specific metabolic markers including dihydrofolate reductase-mediated methotrexate (MTX) and glutamine synthetase-mediated methionine sulfoximine (MSX)-based selection systems.
Although all CHO lineages share the same ancestor, there is significant genetic heterogeneity that exists between these lineages as a result of extensive mutagenesis and clonal selection. To date, the most widely used CHO cell lines include HCO-K1, CHO DXB11, CHO-S, and CHO DG44, all of which exhibit different karyogram and epigenetic variations that contribute to their individual properties.
For example, whereas CHO-K1 cells are associated with better cell-specific productivity that can achieve a production rate within the range of 7 to 16 picograms (pg) of protein/cell/day, the CHO-S cell line is associated with a preference for biomass production, lower Mab expression, and a lower production rate of 2 to 6 pg/cell/day. Importantly, each CHO host will often have different culture conditions that can cause variations to arise in their batch, fed-batch, and semi-continuous perfusion culture methods, as well as the chemically-defined media that is used to facilitate the growth of specific MAbs.
How CHO cells produce MAbs
To date, over 70% of the recombinant proteins that are currently produced by the international biopharmaceutical industrial sector are manufactured using CHO cell technology. In 1997, the first mouse chimeric MAb to obtain approval by the United States Food and Drug Administration (FDA) was rituximab, which is currently approved for the treatment of non-Hodgkin’s lymphoma, chronic lymphocytic leukemia, and rheumatoid arthritis.
Typically, the production of MAbs and proteins from CHO cells begins within the thawing of a suspension CHO cell line that expresses the Mab of interest. These cells are then grown in a specified CHO medium that is often enhanced through the addition of various supplements that are required to produce the protein of interest.
The cultivation of CHO cells can either take place in a shake flask cell culture or through the use of bioreactors. When the shake flask culture method is employed, cell cultures are often performed in triplicate in a 500 milliliter (mL) Erlenmeyer flask. The flasks then remain in a cell culture incubator that shakes at 140 runs per minute (rpm) and is maintained at a carbon dioxide (CO2) humidity level within the range of 5% to 8%.
Comparatively, a bioreactor can also be used to cultivate CHO cells for Mab production. By definition, a bioreactor is any type of manufactured device or system that supports and biologically active environment.
One of the advantages associated with the use of a perfusion bioreactor as compared to the shake flask cell culture method is that cells can be cultured over much longer periods as compared to conventional flasks. This can be achieved by continuously feeding the cells with fresh media and then removing any media that has been fully used. Furthermore, antibodies can be continuously harvested and purified from bioreactors, thereby improving the quality and production rate of MAbs through this approach.
MAb purification
One popular method of MAb purification is through the use of an automated protein A fast protein liquid chromatography (FPLC) method. Here, a protein A column is added to the benchtop FPLC system that consists of a pump, ultraviolet (UV) detector, conductivity meter, and fraction collector. Once the cell medium is injected into the FPLC, medium pressure chromatography is used to separate the proteins according to their size and charge. During the size-dependent separation process, the protein A column ensures that only proteins of a specific size are allowed to pass onto the fraction collector.
Another common MAb purification method is through the use of Protein A/G affinity chromatography. First, the cell culture media sample is filtered, typically through the use of a 0.45 micrometer (µm) filter, to remove the cells, cell debris, lipids, and any other unwanted material from the sample. The filtered sample is then allowed to pass through a filter, wherein the antibodies of the sample bind to the Protein A/G in order to obtain a final sample with a purity that generally exceeds 80%.
Although several other methods, such as ion exchange and gel chromatography, are available to purify antibodies, they are generally not preferred, as these processes often require extensive steps. However, antibodies that are particularly sensitive to certain conditions like specific pH ranges may need to be purified through these alternative methods.
Sources:
- Li, F., Vijayasankaran, N., Shen, A., et al. (2010). Cell culture processes for monoclonal antibody production. MAbs 5; 466-477. doi:10.4161.mabs.2.5.12720.
- Quinteros, D. A., Bermudez, J. M., Ravettin, S., et al. (2017). Therapeutic use of monoclonal antibodies: general aspects and challenges for drug delivery. Nanostructures for Drug Delivery; 807-833. doi:10.1016/B978-0-323-46143-6.00025-7.
- Goey, C. H., Bell, D., & Kontoravdi, C. (2019). CHO cell cultures in shake flasks and bioreactors present different host cell protein profiles in the supernatant. Biochemical Engineering Journal 144; 185-192. doi:10.1016/j.bej.2019.02.006.
- Velugula-Yellela, S. R., Powers, D. N., Angart, P., et al. (2019). Purification and Analytics of a Monoclonal Antibody from Chinese Hamster Ovary Cells Using an Automated Microbioreactor System. Bioengineering. doi:10.3791/58947.
- Purification of Monoclonal Antibodies by Protein A/G column. [Online]. Available from: www.denovobiotech.com/.../purification-of-monoclonal-antibodies-by-protein-ag-column.
Further Reading