A peptidomimetic is a small molecule designed to be structurally and chemically similar to a naturally occurring peptide, thereby mimicking its biological function. Many peptidomimetics are produced by modification of existing peptides, tailored to improve stability and function, or otherwise constructed from small molecule analogs that maintain the structure-activity relationship of the parent peptide.
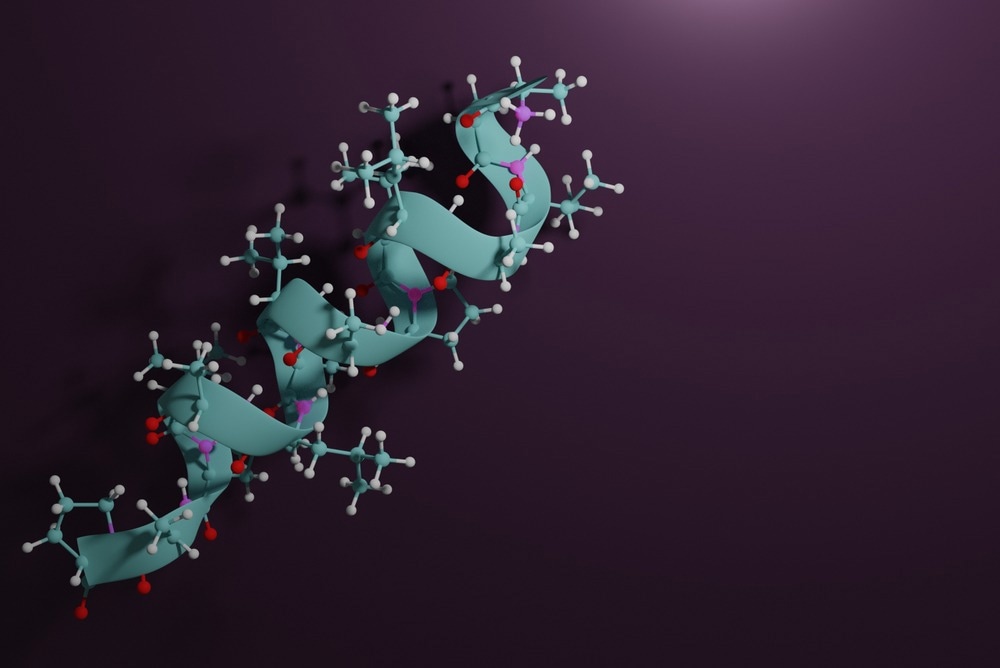
Image Credit: sanjaya viraj bandara/Shutterstock.com
The therapeutic potential of peptidomimetics is investigated in pharmaceutical research just as any other small molecule drug, and structure-activity relationships between a peptide and the target site can be investigated by in silico and in vitro methods just as in fragment-based drug discovery. Subsequent modifications to the chemical and steric structure of the peptide can then be performed to optimize target site affinity and drug-likeness.
Peptides commonly act as messenger and signaling molecules in and between cells, interacting with specific receptors to induce a biochemical response that may promote or inhibit protein activity. As drug molecules, peptides suffer from several drawbacks. However, they generally exhibit poor pharmacokinetic properties owing to their relatively large molecular weight and a large number of proton donor and acceptor groups, interacting widely with various enzymes and other proteins in the body to promote off-target effects and being rapidly excreted during circulation.
Modifying existing peptides or synthesizing non-peptide peptidomimetics to improve drug-likeness, therefore, exploits the existing structure-activity relationship between peptide and target while producing a biocompatible compound that can be safely administered and will survive circulation for long enough to reach the target site.
How are peptidomimetics developed?
Once the structure-activity relationship between a peptide and protein is observed, then researchers can make modifications to the peptide and test the response to uncover the most essential structural and chemical features that enable bonding, though if a starting structure is completely unknown, then a large library of peptides and peptidomimetics may be explored as potential lead compounds in a fragment-based drug discovery approach.
The functionality of each amino acid belonging to the peptide is first identified, finding which binding sites actually promote or reduce affinity for the target. This may be done by individual amino acid replacement in the peptide chain, producing a library of similar peptides that differ by a single amino acid and can be tested individually. As discussed, the large molecular weight of peptides limits their drug-likeness, and thus truncation of the peptide is often performed wherein the shortest sequence possible that still maintains biological activity is explored by removal of amino acids or replacement with shorter ones. Methylation of the peptide may also be performed to block hydrogen bonding, helping researchers to realize which anchor points are most important to affinity towards the target.
One method of promoting peptidomimetic stability and good functional group orientation is macrocyclization. Otherwise, flexible linear peptides are replaced with cyclic structures that do not bend or rotate relative to their functional groups and thus maintain their arrangement permanently. Various synthetic strategies may be employed to perform macrocyclization on linear peptides. These generally involve creating bonds between side chains or head and tail groups to create one or more, usually five – or six-membered rings.
Peptidomimetics are divided into four classes that indicate how they are constructed: those in class A are most similar to their parent peptide, carrying only modifications that improve stability to ensure that the peptide reaches its target without early excretion and in the correct conformation. Class B peptidomimetics are further modified by introducing non-natural amino acids or other small molecule building blocks, often having undergone major alterations to the peptide backbone. The spatial arrangement of side chains and functional groups is maintained to ensure continued affinity towards the target site, though these changes can greatly enhance drug-likeness and circulation time in the body.
Class C peptidomimetics have had their entire peptide backbone replaced with small molecule alternatives that again maintain the spatial arrangement of essential functional groups belonging to the peptide but exhibit enhanced drug-likeness. Finally, class D peptidomimetics bear the least resemblance to their peptide parent and are generally small molecule drugs that interact with the same target site as the parent protein and perform the same biological function. These peptidomimetics are usually generated via high throughput screening of expansive chemical libraries to discern structure-activity relationships rather than from successive generations of peptide modification and testing.
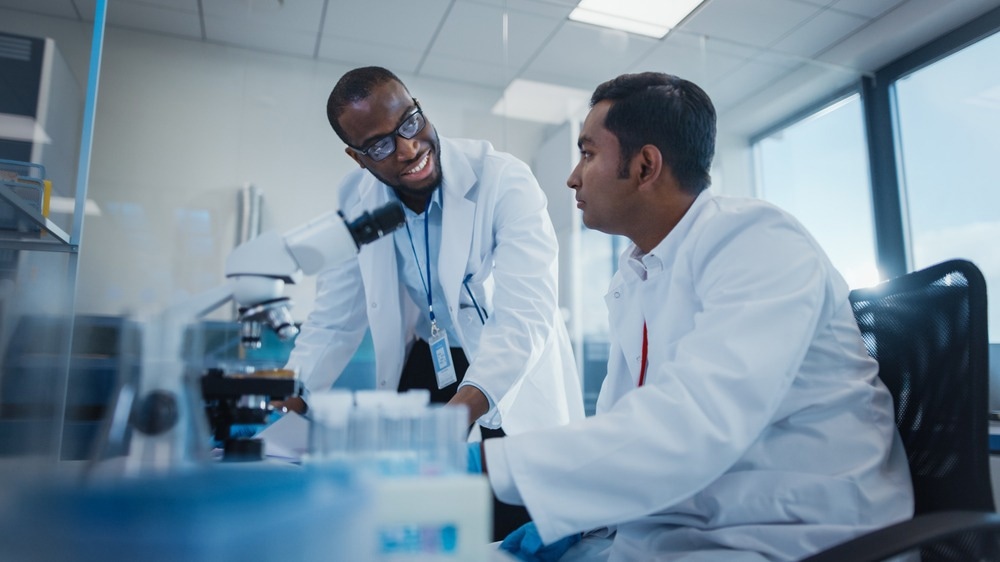
Image Credit: Gorodenkoff/Shutterstock.com
What are some examples of successful peptidomimetics?
Over 20 new peptide or peptidomimetic compounds have been approved by the FDA between 2015 and 2021, representing around 5% of all novel drugs released into the market in this time frame.
One example of a successful peptidomimetic is macrimorelin acetate, approved in 2017 for diagnosing adult growth hormone deficiency and based on a modification of growth hormone realizing tripeptide EP-51389. The molecule consists of a central D-tryptophan amino acid and a modified C-terminus residue that is methylated to prevent hydrogen bonding interactions and encourage the adoption of cis functional groups, improving drug-likeness and specificity to the target site. The salt formulation of this drug can also be delivered orally, which is advantageous over the otherwise employed intravenously delivered diagnostic compounds. Once the target site in the pituitary gland is reached, the peptidomimetic causes the secretion of growth hormone in healthy individuals, which can be detected in the blood.
Broad-spectrum antiviral activity is also reported from some peptidomimetics, which bind strongly with highly conserved regions of the viral proteome and inhibit viral function. For example, severe acute respiratory syndrome coronavirus (SARS-CoV), middle eastern respiratory syndrome coronavirus (MERS-CoV), and SARS-CoV-2 all share the MPRO enzyme that is essential to viral replication, and thus several peptides that block active sites or prevent structural rearrangement of the protein during its normal function are capable of inhibiting viral activity. Several α-ketoamide peptidomimetics have shown good efficacy in inhibiting SARS-CoV-2 viral activity, with those carrying what is known as aldehyde “warheads” demonstrating the most effective inhibition. Electron-rich functional groups such as an aldehyde may form a strong covalent bond with a specific target atom around the binding site and make inhibition much longer lasting, dramatically improving the effectiveness of peptide therapy.
References:
- Li Petri, G., Di Martino, S., & De Rosa, M. (2022). Peptidomimetics: An Overview of Recent Medicinal Chemistry Efforts toward the Discovery of Novel Small Molecule Inhibitors. Journal of Medicinal Chemistry, 65(11), 7438–7475. https://doi.org/10.1021/acs.jmedchem.2c00123
- Lenci, E., & Trabocchi, A. (2020). Peptidomimetic toolbox for drug discovery. Chemical Society Reviews, 49(11), 3262–3277. https://doi.org/10.1039/d0cs00102c
- Gehringer, M., & Laufer, S. A. (2019). Emerging and Re-Emerging Warheads for Targeted Covalent Inhibitors: Applications in Medicinal Chemistry and Chemical Biology. Journal of Medicinal Chemistry, 62(12), 5673–5724. https://doi.org/10.1021/acs.jmedchem.8b01153
Further Reading