Current cancer treatments
There are multiple forms of cancer treatments available to us today, the most common being cytotoxic chemotherapy, radiotherapy, or surgery. While surgery aims to remove the physical tumor from the body, chemotherapy and radiotherapy use anti-cancer drugs, external x-rays, or internal radioactive liquids to target replicating DNA within malignant cells to cause cell-cycle arrest.
Although these treatments prove success in killing cancer cells, they do not specifically target them. The cytotoxic drugs cause DNA damage in healthy cells too, which devises a plethora of negative side effects. Thus, scientists are working to improve cancer treatments by developing drugs that target proteins that are specifically overexpressed in certain types of cancer: an example is creating HSP90 inhibitors.
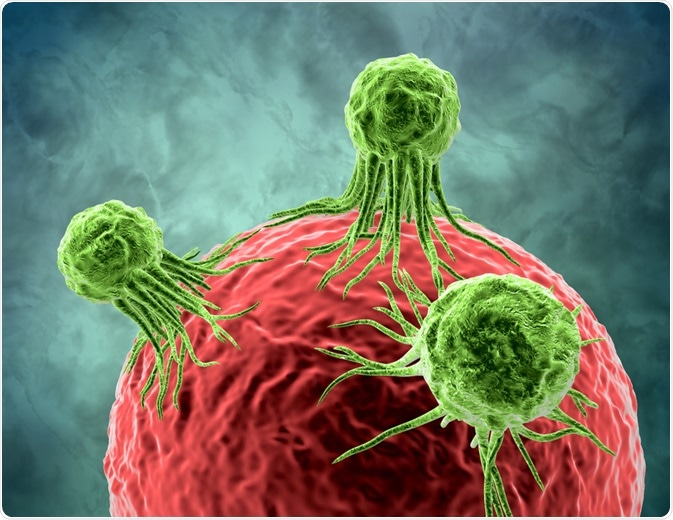
Cancer Cells. Image Credit: Illustration Forest/Shutterstock.com
HSP90 and its client proteins
Heat shock protein 90 is a molecular chaperone that is vital for cellular survival during stressful conditions such as radiation, extreme temperatures, exposure to toxins, etc. It prevents misfolding of a variety of client proteins essential for cell proliferation, ensuring the correct 3D structure of these proteins are maintained.
HSP90 exists as a homodimer with an N terminal domain that binds ATP. Binding causes dimerization and activation of HSP90, causing ATP hydrolysis to form ADP and Pi, inducing the separation of N terminal domains. Then, HSP90 is ready to activate and stabilize client proteins.
HSP90’s client proteins are essential for overall cell survival, growth, and proliferation, but are over-expressed in certain cancers. Consequently, competitive inhibitors for HSP90’s ATP binding sites have been created to aid the degradation and destabilization of client proteins through the proteasome.
Some examples of HSP90’s client proteins are:
- HER2/ERBb2 = A member of the EGFR family, which is a family of receptor tyrosine kinases located on cells’ plasma membrane. Upon ligand binding, these receptors dimerize and activate downstream signaling proteins – ultimately leading to cell proliferation, growth, survival, migration, etc. HER2 is often up-regulated in breast cancer.
- RAF1 = A kinase molecule downstream of receptor tyrosine kinases. RAF1 is a part of the MAPK signaling pathway, downstream of which leads to cell growth and survival. RAF1 is also upregulated in breast cancers, as well as oesophageal cancer, colon cancer, and melanomas.
- CDK4 = Cyclin dependant kinase 4 enzyme is encoded by the CDK4 gene and is key for cell cycle progression.
Types of HSP90 inhibitors
Geldanamycin
Geldanamycin is a naturally occurring antimicrobial compound produced by a strain of S. hygroscopicus var. geldanus, discovered in 1970. It was proven to be an HSP90 inhibitor in 1994 as it undergoes competitive inhibition of HSP90's N terminus causing degradation of its client proteins.
Although Geldanamycin had shown success against specifically malignant cells both in vivo and in-vitro, it has demonstrated many side effects such as severe hepatotoxicity and poor solubility in pre-clinical species. Due to this, Geldanamycin could not progress in clinical trials.
Fortunately, alternative semi-synthetic analogs of Geldanamycin with improved pharmacokinetic profiles were developed and are in clinical studies today. The two main semi-synthetic HSP90 inhibitors that will be discussed in this article are 17AAG and 17DMAG.
17AAG
Unlike Geldanamycin, 17AAG is highly soluble in water and showed promising anti-cancerous effects in vitro and in vivo. It also showed some success in combination with already existing treatments. 17AAG was shown to reduce the expression of p185 in lung cancer cells when used in combination with Paclitaxel. It also showed success in combination with monoclonal antibody Trastuzumab against HER2 positive breast cancer.
Although showing a 59% clinical benefit in patients with pre-treated Trastuzumab, the client proteins rebounded within 72 hours. To defeat this, a toxic level of 17AAG would be required which cannot be ethically given in a clinical trial. 17AAG was also difficult to formulate and therefore expensive to produce, plus complicated to administer pharmacologically.
Consequently, second-generation HSP90 inhibitors such as 17DMAG were produced. These compounds have oral formulations, therefore, have a higher maximum tolerated dose and can be administered to patients more frequently to overcome rebounding of client proteins.
17DMAG
Similar to 17AAG, 17DMAG also binds to the N terminus of HSP90. 17DMAG showed additional benefits such as improved bioavailability and less toxic metabolites. In vivo, 17DMAG was shown to specifically bind to tumor cells and inhibit growth in various cancer types such as Leukaemia, melanoma, breast cancer, ovarian cancer, multiple myeloma, liver cancer, and many more. Some of the client proteins successfully degraded by 17DMAG as a result of HSP90 inhibition were: HIF1, VEGF, STAT3, HER2, mTOR.
New and improving nano-technology improves the transport of 17DMAG to the desired location via intracellular administration using Polymeric nanoparticles. This technology carries the potential to reduce the side effects and increase the clinical efficacy of 17-DMAG. These improvements in the administration of the drug contribute towards entering 17DMAG in more combination therapy-based phase 1 and 2 clinical trials, and it is hoped that this form of targeted therapy will be available in the future.
Conclusion
HSP90 proteins allow the correct folding of proteins, known as client proteins, that are up-regulated in various types of cancer. With competitive inhibition of the ATP binding site, suppressing HSP90 has shown to have therapeutic effects in different cancer types with minimal side effects or death of healthy cells.
17AAG and 17DMAG are examples of semi-synthetic HSP90 inhibitors that have shown positive results in killing targeted cancer cells, with a clear indication of prospects such as combination therapy to enhance the therapeutic effect, and nano-technology to improve specificity for HSP90.
Targeting HSP90 is therefore likely to push towards a positive outcome in cancer treatment, as phase 2/3 clinical trials progress with successful results against cancers such as breast, lung, NSCLC, and many more.
Sources:
- Pearl, L. (2016). Review: The HSP90 molecular chaperone-an enigmatic ATPase. Biopolymers, 105(8), pp.594-607.
- Prodromou, C (2017). Structural Basis of Biological function HSP90 lecture notes. University of Sussex [date accessed: 29th December 2018)
- Whitesell, L., Mimnaugh, E., De Costa, B., Myers, C. and Neckers, L. (1994). Inhibition of heat shock protein HSP90-pp60v-src heteroprotein complex formation by benzoquinone ansamycins: essential role for stress proteins in oncogenic
- Nguyen, D., Chen, A., Mixon, A. and Schrump, D. (1999). Sequence-dependent enhancement of paclitaxel toxicity in non–small cell lung cancer by 17-allylamino 17-demethoxygeldanamycin. The Journal of Thoracic and Cardiovascular Surgery, 118(5), pp.908-915.
- Modi, S., Stopeck, A., Linden, H., Solit, D., Chandarlapaty, S., Rosen, N., D'Andrea, G., Dickler, M., Moynahan, M., Sugarman, S., Ma, W., Patil, S., Norton, L., Hannah, A. and Hudis, C. (2011). HSP90 Inhibition Is Effective in Breast Cancer: A Phase II Trial of Tanespimycin (17-AAG) Plus Trastuzumab in Patients with HER2-Positive Metastatic Breast Cancer Progressing on Trastuzumab. Clinical Cancer Research, 17(15), pp.5132-5139.
- Egorin, M., Lagattuta, T., Hamburger, D., Covey, J., White, K., Musser, S. and Eiseman, J. (2002). Pharmacokinetics, tissue distribution, and metabolism of 17-(dimethylaminoethylamino)-17-demethoxygeldanamycin (NSC 707545) in CD 2 F 1 mice and Fischer 344 rats. Cancer Chemotherapy and Pharmacology, 49(1), pp.7-19.
- Mellatyar, H., Talaei, S., Pilehvar-Soltanahmadi, Y., Barzegar, A., Akbarzadeh, A., Shahabi, A., Barekati-Mowahed, M. and Zarghami, N. (2018). Targeted cancer therapy through 17-DMAG as an Hsp90 inhibitor: Overview and current state of the art. Biomedicine & Pharmacotherapy, 102, pp.608-617.
Further Reading