Hungarian agricultural engineer Karl Ereky coined the term 'biotechnology' in 1919.1 Fermentation is a crucial element of biotechnology and this process is used to create a wide range of key products across a number of industries, including:
- Biomaterials: biodegradable, energy-efficient plastics
- Pharmaceuticals: prophylactics, vaccines, antibiotics, hormones such as human insulin
- Animal nutrition: amino acids, feed supplements
- Bioenergy: bioalcohol fuels based on low value, non-food based feedstocks
Recent years have seen online process analytical technology (PAT) has become increasingly popular in the biotechnology industry.
A number of fermentation scientists have been employing Thermo Scientific™ process mass spectrometers as PAT tools since the early 1980s. These tools are able to precisely and rapidly monitor the composition of gas streams into and out of fermenters and bioreactors.
Other essential fermentation products include vitamins, food additives and industrial enzymes.
Fermentation
The term ‘fermentation’ is employed by microbiologists when describing the production of a product using the mass culture of a microorganism. The product in question may be a foreign product, the microorganism’s own metabolite, or even the cell itself (biomass production).
Microorganisms that perform their metabolism via oxygen are referred to as aerobic microorganisms. A number of microorganisms exist that are able to substitute nitrate or sulfate for oxygen, allowing them to grow in the absence of oxygen. These are referred to as anaerobic microorganisms.
Types of fermentation process
Fermentation processes fall into three common types. These are outlined below.
Batch fermentation
A sterilized nutrient solution is inoculated with microorganisms before being incubated in the fermenter. If the microorganisms are aerobic, oxygen will be added along with acid or base to control the pH during the fermentation.
Cell activity will generally result in constant changes in biomass concentration, metabolite concentration and composition of the culture medium.
Fed-batch fermentation
This process is an enhancement of the closed batch process. Here, a substrate is added in increments as the fermentation progresses.
Continuous fermentation
An open system is put in place, with sterile nutrient solution added to the bioreactor on a continuous basis while an equivalent amount of converted nutrient solution with microorganisms is simultaneously harvested off the system.
Both bioreactors and fermenters have historically been designed to be utilized repeatedly, meaning that it was necessary to carefully sterilize the vessel between batches in order to avoid possible contamination.
More recently, single-use vessels have become common, therefore eliminating the need to manage costly and time-consuming sterilization schedules.
Figure 1 displays a HyPerforma™ 50 liter Single-Use Bioreactor (S.U.B.) from Thermo Scientific™.
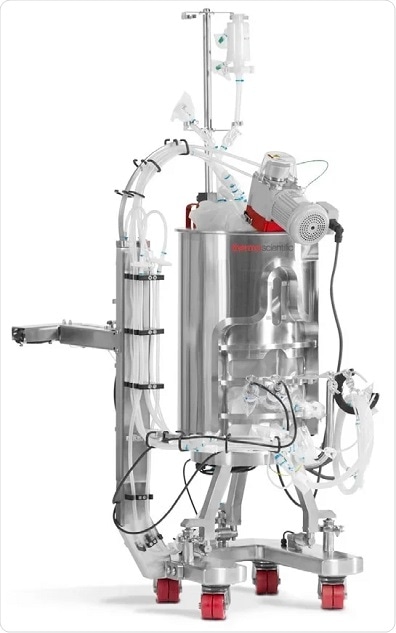
Figure 1. Thermo Scientific™ HyPerforma™ 50 L Single-Use Bioreactor (S.U.B.). Image Credit: Thermo Fisher Scientific – Environmental and Process Monitoring Instruments
While this particular instrument is employed in mammalian cell culture fermentation, it is also available in 100, 250, 500, 1000 and 2000 liter models, ensuring consistent scalability across a wide range of application usages.
Single-Use Fermentors (S.U.F.) of 30 and 300-liter sizes are also available from Thermo Scientific™ and these are ideal for use in fungal or bacterial fermentation.
There are three types of host organism suitable for fermentation processes:
Microbial
Microbial organisms suitable for fermentation processes include molds and yeasts such as Saccharomyces cerevisiae (Brewers’ yeast). These microorganisms are considered to be safe and have therefore been in use for a number of years.
This makes product approval using microbial organisms easier and they are able to produce high product volumes relatively inexpensively.
Bacteria
Bacteria suitable for fermentation processes include Bacillus, E coli and lactic acid bacteria. These bacteria offer a number of benefits, including fast growth, high cell densities and cheap substrates.
They are relatively easy to cultivate and scale up, but a large number of these bacteria are considered to be unsafe as pathogens. This can make product recovery much more challenging.
Mammalian
Mammalian organisms suitable for fermentation processes include Chinese Hamster Ovary (CHO) cells and hybridomas. These organisms can generate highly dedicated products in active, highly specific forms, for example, vaccines, interferons, monoclonal antibodies and recombinant therapeutic proteins.
These organisms require more complex production routes than microbial and bacterial processes. The cell lines are less robust, as well as being more sensitive to shear and stress.
Thermo Scientific™ Gas Analysis Mass Spectrometers have been utilized with microbial and bacterial processes for over 30 years. More recently, interest has grown in mammalian cell culture as these offer the potential for revolutionary advances in vaccines, monoclonal antibodies and gene therapy.
While the requirements of mammalian cell fermentation processes pose distinct challenges for an off-gas analyzer, mass spectrometers from Thermo Scientific™ have already proven to be invaluable in increasing yields and improving the understanding of mammalian cell cultures.
The need for gas analysis
It is essential that the state of the culture in any fermentation be monitored because it is the health of this culture that governs the conversion rate of nutrients, the formation of unwanted by-products and, in the worst case scenario, the onset of poisoning.
Analysis of respiratory gases being fed into and removed from the fermenter offers an ideal means of characterizing the fermentation process.
This non-invasive approach allows effective monitoring of the fermentation’s physiological state, including substrate consumption and growth kinetics. It can also help determine the most effective point at which to halt the process in order to ensure maximum yield.
Why use mass spectrometry for gas analysis?
Several fermentations are characterized by tiny alterations in carbon dioxide and oxygen concentrations at crucial phases of the fermentation, for example, during the lag phase when the nutrients and microorganisms exist in equilibrium.
It is, therefore, vital that the technique employed for measuring off-gas is capable of rapid, accurate analysis in order to capture these subtle changes.
The speed of MS makes it ideal for these types of fermentation applications, but it is important that speed is not at the expense of accuracy - precise data collection is also essential. Otherwise, the method will fail to record small changes in concentration.
There are two common, untrue assumptions surrounding fermentation applications. The first is that only the quantification of carbon dioxide and oxygen in off-gas is necessary when making the first steps towards process control.
The second is that it is possible to attain sufficient accuracy through discrete measurement technology alone.
External biology will result in ongoing variance in the sparge gas. Animals and humans undergo respiration, consuming O2 and generating CO2, but plant photosynthesis also consumes CO2 while producing O2 during daylight hours.
Twin-tower desiccant dryer systems will either regurgitate or absorb CO2 depending on where they currently are within the regeneration cycle.
Figure 2 summarizes these influences, displaying an example of day and night variations in sparge gas levels of CO2 and O2. These measurements were taken over a 24 hour period using a Prima PRO process mass spectrometer.
Accurate pre-screening for potential contamination can only be achieved through an accurate comparison of sparge gas and effluent gas. Accurate comparison is also necessary in order to provide real-time information around culture respiration and the availability of nutrients.
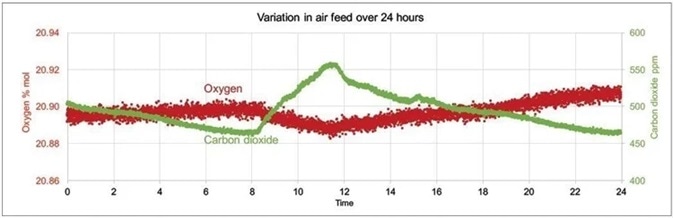
Figure 2. Example of day and night variations in sparge gas levels of CO2 and O2 measured with Prima PRO over 24 hours. Image Credit: Thermo Fisher Scientific – Environmental and Process Monitoring Instruments
Benefits of magnetic sector MS
Two types of MS have been utilized in order to monitor fermentation processes. These are quadrupole, which sees charged particles separated in a variable RF field and magnetic sector, which sees charged particles separated in a variable magnetic field.
Thermo Scientific™ has more than thirty years’ industrial experience in the production of both quadrupole and magnetic sector mass spectrometers. Rigorous testing has determined that the magnetic sector-based analyzer offers the best performance for fermentation off-gas analysis.2,3
Magnetic sector analyzers offer a number of key benefits, including improved precision, accuracy, resistance to contamination and long intervals between required calibrations.
Analytical precision is generally between two and ten times better than a quadrupole analyzer, depending on the complexity of the mixtures and the gases under analysis.
Signal intensity is shown as a flat top peak at any specific position on a magnetic sector analyzer. This means that a minor drift in the mass scale will not result in an alteration in signal intensity. This is not the case with quadrupole mass spectrometers, as these supply rounded peaks.
The magnetic sector analyzers employed in Thermo Scientific’s Prima range of mass spectrometers are laminated, allowing them to scan at speeds equivalent to those of quadrupole analyzers while providing a distinct combination of high stability and rapid analysis.
This method enables the rapid and exceptionally stable analysis of an unlimited number of user-defined gases. Table 1 confirms the outstanding long-term stability of magnetic sector MS.
Table 1. Analysis of compressed air cylinder over 7 days by Prima BT magnetic sector MS. Source: Thermo Fisher Scientific – Environmental and Process Monitoring Instruments
|
Nitrogen %mol |
Oxygen %mol |
Argon %mol |
Carbon dioxide ppm |
Mean |
Std dev |
Mean |
Std dev |
Mean |
Std dev |
Mean |
Std dev |
Day 1 |
78.0807 |
0.0028 |
20.9459 |
0.0026 |
0.9337 |
0.0003 |
396.84 |
1.31 |
Day 2 |
78.0767 |
0.0023 |
20.9494 |
0.0023 |
0.9342 |
0.0003 |
397.46 |
1.25 |
Day 3 |
78.0761 |
0.0024 |
20.9500 |
0.0023 |
0.9342 |
0.0003 |
397.34 |
1.28 |
Day 4 |
78.0798 |
0.0023 |
20.9469 |
0.0023 |
0.9337 |
0.0003 |
396.31 |
1.31 |
Day 5 |
78.0777 |
0.0030 |
20.9487 |
0.0028 |
0.9339 |
0.0003 |
396.76 |
1.34 |
Day 6 |
78.0741 |
0.0023 |
20.9518 |
0.0022 |
0.9344 |
0.0003 |
397.47 |
1.27 |
Day 7 |
78.0750 |
0.0023 |
20.9512 |
0.0022 |
0.9342 |
0.0003 |
397.23 |
1.30 |
In the example presented here, a Prima BT benchtop gas analysis mass spectrometer was configured to analyze oxygen, nitrogen, argon and carbon dioxide in a cylinder of compressed air. This analysis was performed continuously for seven days, with no interruption or recalibration.
The analysis time was set to 5 seconds. Day-to-day mean values for carbon dioxide were found to vary by 1 ppm or less, while day-to-day mean values for nitrogen and oxygen were found to vary by 0.005%.
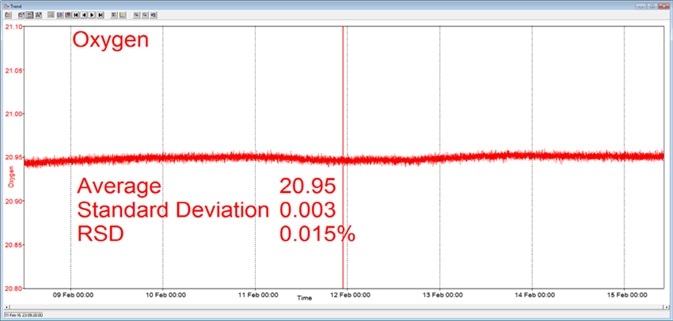
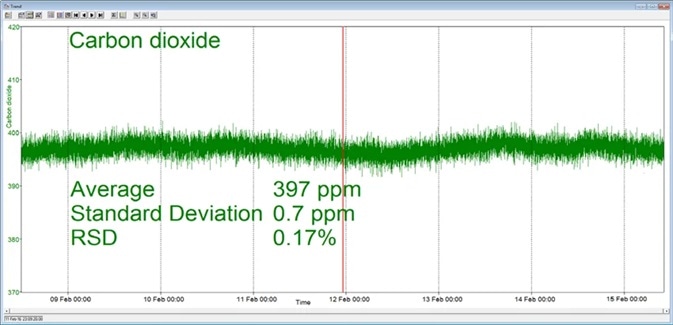
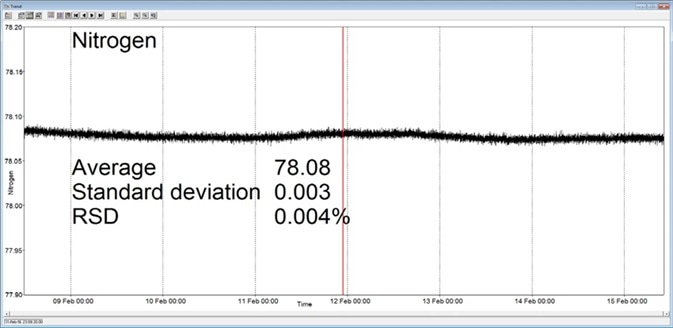
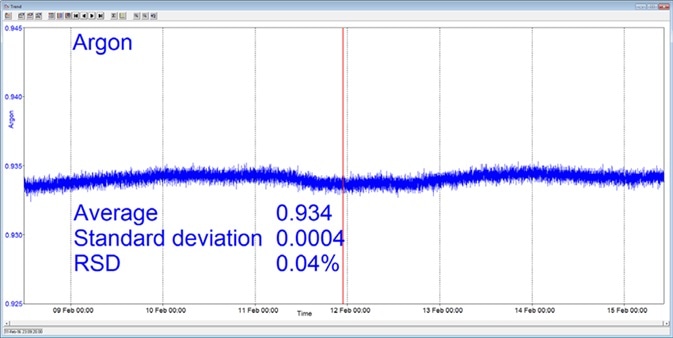
Figure 3. Long-term stability data from Prima BT magnetic sector MS. Image Credit: Thermo Fisher Scientific – Environmental and Process Monitoring Instruments
Rapid multistream sampling
Monitoring multiple bioreactors using MS requires a fast, reliable means of switching between streams. However, rotary valves suffer from poor reliability, while solenoid valve manifolds include too much dead volume.
Thermo Scientific™ has developed the unique Rapid Multistream Sampler (RMS) to address these issues.
The instrument provides an unparalleled combination of sampling speed and reliability, facilitating sample selection from up to 64 streams. Stream settling times are application-dependent and fully user-configurable and the RMS offers digital sample flow recording for each individual stream selected.
This feature can be employed to trigger an alarm if the sample flow drops; for example, should a filter in the sample conditioning system become blocked. The RMS is also heated, ensuring a fast response to even the most ‘sticky’ volatiles.
Respiratory quotient
Respiration is the term used to describe the process whereby an organism oxidizes food to produce energy. The Respiratory Quotient (RQ) is an essential control parameter within the fermentation process.
RQ is the ratio of the Carbon Dioxide Evolution Rate (CER) to the Oxygen Uptake Rate (OUR). Table 2 shows the complete calculation of RQ.
Table 2. The respiratory quotient for fermentation off-gas analysis. Source: Thermo Fisher Scientific – Environmental and Process Monitoring Instruments
CER
(CO2 Evolution Rate) |
= |
(%Volume of CO2 out × flow out) |
- |
(%Volume CO2 in × Flow in) |
OUR
(O2 Uptake Rate) |
= |
(%Volume of O2 in × flow in) |
- |
(%Volume O2 out x Flow out) |
RQ
(Respiratory Quotient) |
= |
CER/OUR |
|
|
A high RQ confirms the generation of high levels of CO2, indicating that the metabolism is working at high efficiency. RQ is also an invaluable means of identifying and controlling the carbon source currently being metabolized.
For example, the RQ would theoretically be one if the carbon source is comprised of glucose or other carbohydrates.
C6H12O6 + 6O2 → 6CO2 + 6H2O
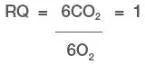
Production of biomass or product formation is not covered in this equation; it only relates to combustion. As such, the RQ for glucose is unlikely to be 1.00; it may actually be approximately 1.04.
Accurate determination of RQ depends on successfully determining the ratio of the flows in and out of the bioreactor. Thankfully, scanning MS can easily determine this ratio because it is able to measure N2 and Ar as well as O2 and CO2.
At least one of these two gases will be inert to the process, meaning that it can be utilized to correct for any humidity change occurring as the dry air feed gas is bubbled through the fermentor liquid.
Without this correction, errors will be introduced into the headspace data due to dilution by the additional water vapor.4 Table 3 includes the RQ calculation utilizing nitrogen as the flow correction. Thermo Scientific’s GasWorks® software is able to calculate RQ as a standard feature for a fermentation application.
Table 3. Respiratory Quotient calculated by MS. Source: Thermo Fisher Scientific – Environmental and Process Monitoring Instruments
= |
{CO2out |
x |
(N2in / N2out)} |
– |
CO2in |
_________________________________________________________________________________________________________ |
|
O2in |
– |
{O2out |
x |
(N2in / N2out )} |
Figure 4 displays data from an E. coli fed-batch fermentation. Thermo Scientific’s MS can be used to measure oxygen, carbon dioxide, nitrogen and argon in both inlet and outlet, as well as being able to calculate RQ.
A reagent is introduced to induce cells at temperature To in order to change from multiplying to expressing the desired product.
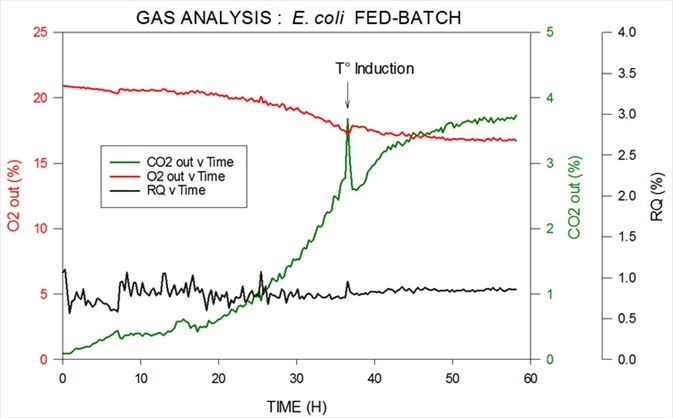
Figure 4. Off-gas and RQ data generated by MS from E. coli fed-batch fermentation. Image Credit: Thermo Fisher Scientific – Environmental and Process Monitoring Instruments
Analysis of volatiles
Respiratory gases are not the only species of interest to be found in off-gas. Volatile organics such as ethyl acetate, ethanol, methanol and even hydrogen sulfide can be found at ppm levels in the headspace and analysis of these volatile organics can offer critical information on the fermentation’s well-being.
Analysis of volatile organics poses some technical problems, however and these must be overcome if the analytical data is to be useful and meaningful. For example, measurement of trace levels of methanol and ethanol requires measurement of the CH2OH+ fragment at mass 31.
It should be noted that there is a very large peak at mass 32 from the percentage levels of O2 in the vent gas. The tail from the 32 peak should be corrected in order to perform an accurate measurement of trace alcohol levels.
Intensity of the tail from O2 at mass 31 compared with the intensity of the peak at mass 32 is approximately 0.02%. Should the concentration of O2 be around 20%, the signal at mass 31 would be equivalent to around 40 ppm.
This interference is recorded during calibration to enable proper correction in any subsequent analysis.
This interference level on a quadrupole MS is variable and much higher, resulting in excessive uncertainty in low level ethanol measurement - a low level ethanol signal would be effectively ‘buried’ in the noise from the oxygen peak.
The measurement is highly reproducible using a magnetic sector instrument with ethanol and methanol quantifiable with a precision as low as 10 ppm. Figure 5 displays data obtained from an S. cerevisiae fed-batch fermentation.
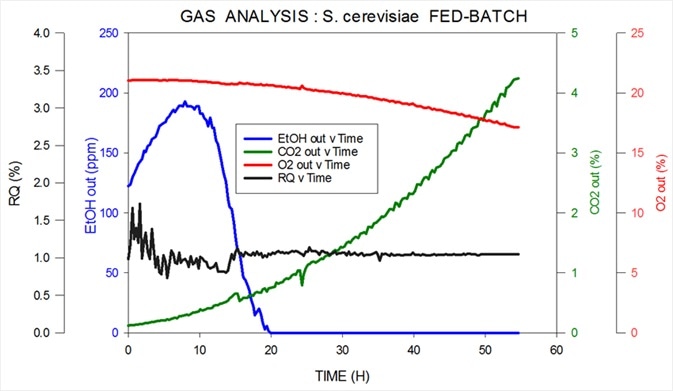
Figure 5. Off-gas and RQ data generated by MS from S. cerevisiae fed-batch fermentation. Image Credit: Thermo Fisher Scientific – Environmental and Process Monitoring Instruments
Thermo Scientific’s MS measures oxygen, carbon dioxide, nitrogen and argon in the inlet while measuring ppm levels of ethanol in the headspace in the outlet.
It also calculates RQ. Ethanol is consumed as the initial carbon source; then, this is switched over to glucose in order to express the desired product.
Because the volume of oxygen consumption is low and the signal-to-noise ratio is correspondingly low, precision is lower at the very start of the fermentation. This period is known as the ‘lag phase,’ where the cell count is extremely low. Precision rapidly improves as the organisms begin to multiply.
Table 4 displays the standard performance specifications for the Prima PRO magnetic sector MS from Thermo Scientific. Over 16 hours, precision is the standard deviation seen. The extremely high precision of 0.05% relative over 16 hours for oxygen should be noted.
Analysis time - including stream switching time - is 30 seconds per stream for all six components. Should methanol and ethanol be removed from the analysis, analysis time decreases to 10 seconds per stream.
Table 4. Example of a standard performance specification for Prima PRO Process MS. Source: Thermo Fisher Scientific – Environmental and Process Monitoring Instruments
Component |
Concentration range
%mol |
The precision of analysis by Prima PRO
(single standard deviation) ≤ |
Nitrogen |
0 – 100 |
0.005 %mol |
Oxygen |
0 – 100 |
0.005 %mol |
Argon |
0 – 1 |
0.001 %mol |
Carbon dioxide |
0 – 10 |
0.1% relative or 0.0003 %mol* |
Methanol |
0 – 1 |
2% relative or 0.001 %mol* |
Ethanol |
0 – 1 |
2% relative or 0.001 %mol* |
*-Whichever is greater
Mammalian cell culture
In bacterial and microbial fermentation, feed gas composition is fairly constant; this is either air or air enriched with oxygen. In mammalian cell fermentation, however, the feed gas composition is a constantly shifting mixture of several compounds, for example, nitrogen, oxygen and carbon dioxide.
Feed gas concentration ranges also vary dramatically, with carbon dioxide varying from tens of parts per million to tens of percent, for example.
Utilization of an overlay gas introduces further complications as the overall inlet gas composition will be comprised of a mixture of overlay gas and sparge gas. Using sodium bicarbonate as a pH buffer may also contribute to carbon dioxide levels in the outlet.
All of these factors contribute to a challenging set of requirements for the off-gas analyzer if the instrument is to provide meaningful online data. This is especially important if it is to effectively complement off-line data such as metabolite concentration and viable cell count data.
Figure 6 provides details on how a magnetic sector’s precision and speed impact its ability to supply this valuable data.
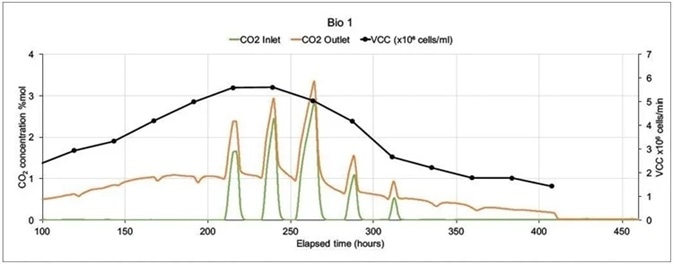
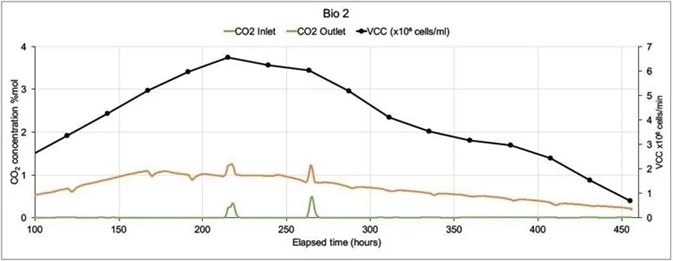
Figure 6. On-line CO2 inlet and outlet data and off-line Viable Cell Count data from two mammalian cell culture bioreactors. Image Credit: Thermo Fisher Scientific – Environmental and Process Monitoring Instruments
In the example presented here, a Prima BT was used to monitor two 5 liter bioreactors in which modified Chinese Hamster Ovary (CHO) cells were being used to express monoclonal antibodies.
The MS analyzed inlet and outlet gases for O2, CO2, N2 and Ar in each bioreactor. Cell count (both viable and total), lactate levels and glucose levels were also analyzed off-line. There was only one inlet for each bioreactor (sparge gas) which fed a combination of O2, CO2, N2 and Air.
Carbon dioxide was utilized to control pH, while oxygen feed was used to control the dissolved oxygen. MS cycle time was 15 seconds per point, including settling time. Concentrations were averaged over 99 readings to allow for a reduction in noise caused by perturbations in sparge gas concentrations.
Figure 6 shows data from 100 hours onwards as there was very little activity at the beginning of the run. Bio 1 exhibits a very different inlet CO2 profile despite both bioreactors being controlled using the same controller. This was because considerable amounts of CO2 were injected to adjust pH.
Bio 2’s more stable CO2 profile led to a higher Viable Cell Count with an extended culture duration. The control strategy was amended in subsequent runs, resulting in extensions in culture durations and increases in VCC levels.
Scale-up from laboratory to bulk production
Manufacturing processes usually commence with cell cultures grown in a laboratory. Cells will be sequentially transferred to increasingly large fermenters, ultimately being moved into production vessels able to hold up to 20000 liters of cells and growth media.
It is imperative that the precise environment required by specific cells to remain healthy and grow is maintained. This requires the collection of precise off-gas analytical data at each stage of the scale-up process, from laboratory to pilot plant and eventual bulk production.
In some applications, it may be necessary to utilize separate MS analyzers in the laboratory and the plant, while in other cases, a single mass spectrometer equipped with an appropriate RMS multi-stream inlet will be able to monitor all of the fermentors.
It is vital that results from the two analyzer platforms correlate if a smooth transition is to be ensured throughout the various stages of scale-up.
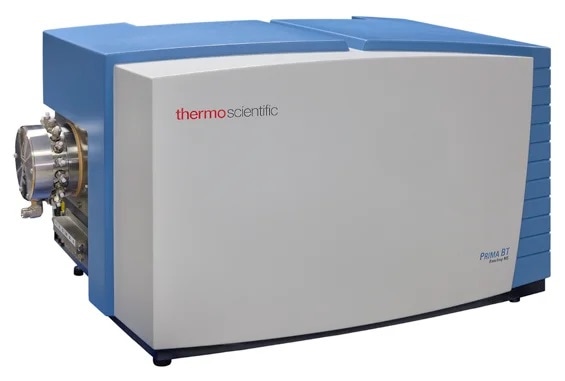
Figure 7. Prima BT process development MS. Image Credit: Thermo Fisher Scientific – Environmental and Process Monitoring Instruments
Figure 7 shows the Prima BT, an example of a mass spectrometer suitable for fermentation process development, while Figure 8 shows the Prime PRO, an example of a mass spectrometer suitable for production process monitoring.
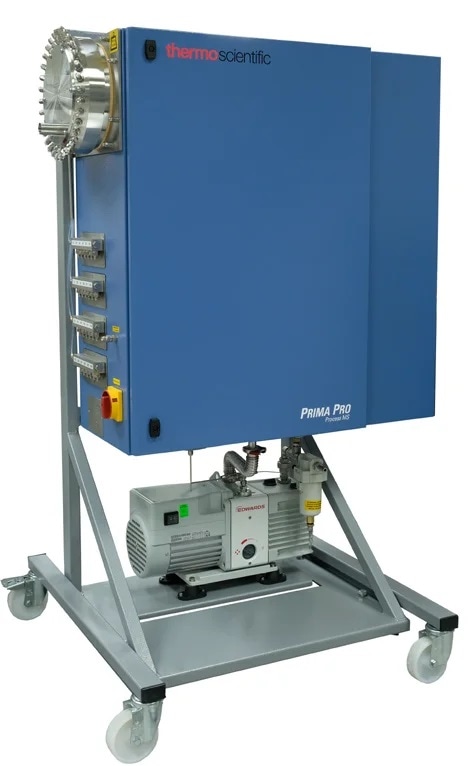
Figure 8. Prima PRO process MS. Image Credit: Thermo Fisher Scientific – Environmental and Process Monitoring Instruments
Each of these two systems utilizes Thermo Scientific’s magnetic sector analyzer for high precision multi-component gas analysis, while each system can also supply the distinctly reliable Rapid Multistream Sampler.
Summary
Magnetic sector mass spectrometers have been shown to offer the highest levels of precision for fermentation off-gas analysis. These instruments have been efficiently monitoring fermentor off-gas at a variety of the world’s leading biotechnology and pharmaceutical companies for a number of years.
The magnetic sector analyzer combines high speed with excellent stability, lending itself ideally to this demanding application.
Thermo Fisher Scientific’s gas analysis MS product range includes the Prima BT and Prima PRO, both of which offer rapid, precise off-gas analysis through each stage of fermentation and cell culture processes, whether these are in a laboratory, pilot plant or during bulk production.
The Prima PRO is able to monitor more than 60 bioreactors with no compromise in sterility, while the Prima BT offers an ideal benchtop solution for smaller-scale bioreactors due to its ability to be configured with 15 samples and six calibration ports.
The comprehensive, extremely precise gas composition measurements provided by each of these models can be incorporated into an APC system with ease. It is possible to achieve significant process control improvements extremely quickly, generally within a day or two of a start-up.
References
- M.G. Fári, U.P. Kralovánszky, The founding father of biotechnology: Károly (Karl) Ereky. Int. J. Hortic. Sci., Vol 12, n.1, p.9-12, 2006.
- David Pollard, Jens Christensen, Vent Gas Analysis, Encyclopaedia of Industrial Biotechnology: Bioprocess, Bioseparation and Cell Technology 2010, John Wiley & Sons.
- Joseph S. Alford, Bioprocess control: Advances and challenges, Computers & Chemical Engineering Volume 30, September 2006.
- P.C. van der Aar, A.H. Stouthamer, H.W. van Verseveld, Possible misconceptions about O2 consumption and CO2 production measurements in stirred microbial cultures, Journal of Microbiological Methods Volume 9, Issue 4, June 1989.
Acknowledgments
Produced using materials originally authored by Graham Lewis from Thermo Fisher Scientific
About Thermo Fisher Scientific – Environmental and Process Monitoring Instruments
We design and manufacture industry-leading products for Gas & particulate pollutants, Flow, gas and liquid measurement, Process analytical measurements, and Industrial Hygiene.
Technologies have proven to help customers improve efficiency, ensure process and quality control, maintain regulatory compliance, and increase worker safety.
Process mass spectrometers
Maximize product yield and increase profitability with process mass spectrometry analysis. Process gas analyzers are engineered to meet a number of challenging process applications in the petrochemical, iron, and steel, and biotechnology industries. Highly reliable and easy-to-own, Thermo Fisher Scientific process gas analysis technologies deliver faster, more complete, lab-quality online gas analysis and process analytics. Learn More
Process Analytical Technology/Biopharma
Process Analytical Technology (PAT) is a regulatory framework initiated by the United States’ Food and Drug Administration (FDA) that encourages pharmaceutical manufacturers to improve the process of pharmaceutical development, manufacturing, and quality control.
PAT aims to improve process efficiency by defining Critical Process Parameters (CPP) and monitoring these CPPs to stay within a defined limit, either in-line or on-line to maintain a product’s Critical Quality Attributes (CQA). Monitoring CPPs with process mass spectrometry gas analysis reduces over-processing, pinpoints contaminants, and increase product consistency. Learn More
Industrial hygiene
Industrial hygiene instruments keep your investments secure, your facilities compliant with local regulations, and your workers safe from leaking pollutants, gases, or toxic vapors. Make sure you have the most updated technology, with access to repair and calibration support to maintain reliable gas monitoring. With our environmental expertise, you can breathe easy knowing your company, workers, and neighboring communities have cleaner, safer, and healthier air quality. Learn More
Sulfur analyzers
Online sulfur analyzers replace expensive and time-consuming laboratory sampling. Get fast responses and wide measurement ranges of trace sulfur and total sulfur in flare gas, liquid, and vapor for reliable emissions monitoring. Learn More
Gas & particulate analyzers
Increasingly stringent regulatory requirements are making it more difficult to maintain regulatory compliance and optimal process performance. Air quality monitoring and reporting requirements in the U.S., China, India, Europe, and Latin America are shifting and being redefined. Together we can arrive at solutions that make sound business sense. Learn More
Flow measurement
Flow measurement and process control are critical aspects of producing, handling, and transporting hydrocarbons around the world. With our Thermo Scientific suite of flow computers and flow meters, we enable our customers to reduce cost, decrease lost material, automate, and monitor critical points in processes. From field to control room and upstream to downstream, our products provide greater control, confidence, and reliability. Learn More
Sponsored Content Policy: AZO Life Science publishes articles and related content that may be derived from sources where we have existing commercial relationships, provided such content adds value to the core editorial ethos of AZO Life Science, which is to educate and inform site visitors interested in medical research, science, medical devices, and treatments.